Carbohydrate metabolism and energy balance -
Supported by a grant from the Academic Network, Portland, Oregon. PDF Split View Views. Cite Cite Peter J. Select Format Select format. ris Mendeley, Papers, Zotero. enw EndNote. bibtex BibTex. txt Medlars, RefWorks Download citation. Permissions Icon Permissions. Close Navbar Search Filter Nutrition Reviews This issue Dietetics and Nutrition Books Journals Oxford Academic Enter search term Search.
Abstract Fructose intake and the prevalence of obesity have both increased over the past two to three decades. obesity , diabetes , cardiovascular disease , triglycerides , leptin.
Issue Section:. Download all slides. Views 1, More metrics information. Total Views 1, Email alerts Article activity alert. Advance article alerts. New issue alert. Receive exclusive offers and updates from Oxford Academic. Citing articles via Web of Science Latest Most Read Most Cited Food bioactive peptides: functionality beyond bitterness.
Perspective on alternative therapeutic feeds to treat severe acute malnutrition in children aged between 6 and 59 months in sub-Saharan Africa: a narrative review.
Exploring the physiological factors relating to energy balance in women with polycystic ovary syndrome: a scoping review. Effects of community-based educational video interventions on nutrition, health, and use of health services in low- and middle-income countries: systematic review and meta-analysis.
More from Oxford Academic. Allied Health Professions. Dietetics and Nutrition. Medicine and Health. About Nutrition Reviews Editorial Board Author Guidelines Contact Us Facebook Twitter YouTube LinkedIn Purchase Recommend to your Library Advertising and Corporate Services Journals Career Network.
Anaerobic muscle enzyme changes after interval training. Sharp, R. Effects of eight weeks of bicycle ergometer sprint training on human muscle buffer capacity. Weston, A. Skeletal muscle buffering capacity and endurance performance after high-intensity interval training by well-trained cyclists.
McKenna, M. Sprint training enhances ionic regulation during intense exercise in men. Gibala, M. Physiological adaptations to low-volume, high-intensity interval training in health and disease.
Lundby, C. Biology of VO 2 max: looking under the physiology lamp. Convective oxygen transport and fatigue. Holloszy, J. Adaptations of skeletal muscle to endurance exercise and their metabolic consequences.
Chesley, A. Regulation of muscle glycogen phosphorylase activity following short-term endurance training. Leblanc, P. Effects of 7 wk of endurance training on human skeletal muscle metabolism during submaximal exercise.
Determinants of endurance in well-trained cyclists. Westgarth-Taylor, C. Metabolic and performance adaptations to interval training in endurance-trained cyclists. Seynnes, O. Early skeletal muscle hypertrophy and architectural changes in response to high-intensity resistance training.
Elevation of creatine in resting and exercised muscle of normal subjects by creatine supplementation. Hultman, E. Muscle creatine loading in men.
Influence of oral creatine supplementation of muscle torque during repeated bouts of maximal voluntary exercise in man. Casey, A. Creatine ingestion favorably affects performance and muscle metabolism during maximal exercise in humans.
Vandenberghe, K. Long-term creatine intake is beneficial to muscle performance during resistance training. Hermansen, L. Muscle glycogen during prolonged severe exercise. Ørtenblad, N. Muscle glycogen stores and fatigue. Matsui, T. Brain glycogen decreases during prolonged exercise.
Diet, muscle glycogen and physical performance. Carbohydrate-loading and exercise performance: an update. Balsom, P. High-intensity exercise and muscle glycogen availability in humans.
Muscle glycogen utilization during prolonged strenuous exercise when fed carbohydrate. Reversal of fatigue during prolonged exercise by carbohydrate infusion or ingestion.
Effect of carbohydrate ingestion on exercise metabolism. Jeukendrup, A. Carbohydrate ingestion can completely suppress endogenous glucose production during exercise. Effect of carbohydrate ingestion on glucose kinetics during exercise.
Nybo, L. CNS fatigue and prolonged exercise: effect of glucose supplementation. Snow, R. Effect of carbohydrate ingestion on ammonia metabolism during exercise in humans. Chambers, E.
Carbohydrate sensing in the human mouth: effects on exercise performance and brain activity. Costill, D. Effects of elevated plasma FFA and insulin on muscle glycogen usage during exercise.
Vukovich, M. Effect of fat emulsion infusion and fat feeding on muscle glycogen utilization during cycle exercise. Odland, L. Effects of increased fat availability on fat-carbohydrate interaction during prolonged exercise in men.
Phinney, S. The human metabolic response to chronic ketosis without caloric restriction: preservation of submaximal exercise capability with reduced carbohydrate oxidation. Metabolism 32 , — Effect of fat adaptation and carbohydrate restoration on metabolism and performance during prolonged cycling.
Havemann, L. Fat adaptation followed by carbohydrate loading compromises high-intensity sprint performance. Decreased PDH activation and glycogenolysis during exercise following fat adaptation with carbohydrate restoration. Low carbohydrate, high fat diet impairs exercise economy and negates the performance benefit from intensified training in elite race walkers.
Paoli, A. The ketogenic diet and sport: a possible marriage. Ketogenic diets for fat loss and exercise performance: benefits and safety? Helge, J. Interaction of training and diet on metabolism and endurance during exercise in man. Yeo, W. Skeletal muscle adaptation and performance responses to once a day versus twice every second day endurance training regimens.
Hulston, C. Training with low muscle glycogen enhances fat metabolism in well-trained cyclists. Kirwan, J. Carbohydrate balance in competitive runners during successive days of intense training. Cox, P.
Nutritional ketosis alters fuel preference and thereby endurance performance in athletes. Shaw, D. Exogenous ketone supplementation and keto-adaptation for endurance performance: disentangling the effects of two distinct metabolic states.
Evans, M. No benefit of ingestion of a ketone monoester supplement on km running performance. Prins, P. Effects of an exogenous ketone supplement on five-kilometer running performance. Dearlove, D. Nutritional ketoacidosis during incremental exercise in healthy athletes. Leckey, J.
Ketone diester ingestion impairs time-trial performance in professional cyclists. Effects of caffeine ingestion on metabolism and exercise performance. Sports 10 , — Graham, T. Performance and metabolic responses to a high caffeine dose during prolonged exercise.
Caffeine ingestion and muscle metabolism during prolonged exercise in humans. Caffeine ingestion does not alter carbohydrate or fat metabolism in human skeletal muscle during exercise. Metabolic, catecholamine, and exercise performance responses to various doses of caffeine.
Desbrow, B. The effects of different doses of caffeine on endurance cycling time trial performance. Sports Sci. Cole, K. Effect of caffeine ingestion on perception of effort and subsequent work production.
Sport Nutr. Kalmar, J. Caffeine: a valuable tool to study central fatigue in humans? Exercise and sport performance with low doses of caffeine. Suppl 2. Wickham, K. Administration of caffeine in alternate forms.
Barnett, C. Effect of L-carnitine supplementation on muscle and blood carnitine content and lactate accumulation during high-intensity sprint cycling.
Stephens, F. Carbohydrate ingestion augments L-carnitine retention in humans. Wall, B. Chronic oral ingestion of L-carnitine and carbohydrate increases muscle carnitine content and alters muscle fuel metabolism during exercise in humans.
Skeletal muscle carnitine loading increases energy expenditure, modulates fuel metabolism gene networks and prevents body fat accumulation in humans. A threshold exists for the stimulatory effect of insulin on plasma L-carnitine clearance in humans.
Larsen, F. Effects of dietary nitrate on oxygen cost during exercise. Bailey, S. Dietary nitrate supplementation reduces the O 2 cost of low-intensity exercise and enhances tolerance to high-intensity exercise in humans. Dietary nitrate supplementation enhances muscle contractile efficiency during knee-extensor exercise in humans.
Lansley, K. Acute dietary nitrate supplementation improves cycling time trial performance. Boorsma, R. Beetroot juice supplementation does not improve performance of elite m runners. Nyakayiru, J.
No effect of acute and 6-day nitrate supplementation on VO 2 and time-trial performance in highly trained cyclists. Jones, A. Dietary nitrate and physical performance. Whitfield, J. Dietary nitrate enhances the contractile properties of human skeletal muscle.
Beetroot juice supplementation reduces whole body oxygen consumption but does not improve indices of mitochondrial efficiency in human skeletal muscle. Dietary inorganic nitrate improves mitochondrial efficiency in humans.
Ntessalen, M. Inorganic nitrate and nitrite supplementation fails to improve skeletal muscle mitochondrial efficiency in mice and humans. Relationship of contraction capacity to metabolic changes during recovery from a fatiguing contraction.
Sutton, J. Effect of pH on muscle glycolysis during exercise. Wilkes, D. Effect of acute induced metabolic alkalosis on m racing time. Acid-base balance during repeated bouts of exercise: influence of HCO 3. Hollidge-Horvat, M. Effect of induced metabolic alkalosis on human skeletal muscle metabolism during exercise.
Street, D. Metabolic alkalosis reduces exercise-induced acidosis and potassium accumulation in human skeletal muscle interstitium. Sostaric, S. Parkhouse, W. Buffering capacity of deproteinized human vastus lateralis muscle.
Derave, W. β-Alanine supplementation augments muscle carnosine content and attenuates fatigue during repeated isokinetic contraction bouts in trained sprinters.
Hill, C. Influence of β-alanine supplementation on skeletal muscle carnosine concentrations and high intensity cycling capacity. Amino Acids 32 , — Powers, S. Exercise-induced oxidative stress: cellular mechanisms and impact on muscle force production.
Merry, T. Do antioxidant supplements interfere with skeletal muscle adaptation to exercise training? Petersen, A. Infusion with the antioxidant N-acetylcysteine attenuates early adaptive responses to exercise in human skeletal muscle.
Ristow, M. Antioxidants prevent health-promoting effects of physical exercise in humans. Natl Acad. USA , — Hyperthermia and fatigue. González-Alonso, J. Dehydration markedly impairs cardiovascular function in hyperthermic endurance athletes during exercise.
Metabolic and thermodynamic responses to dehydration-induced reductions in muscle blood flow in exercising humans. Fink, W. Leg muscle metabolism during exercise in the heat and cold. Febbraio, M. Muscle metabolism during exercise and heat stress in trained men: effect of acclimation.
Blunting the rise in body temperature reduces muscle glycogenolysis during exercise in humans. Influence of body temperature on the development of fatigue during prolonged exercise in the heat. Effect of fluid ingestion on muscle metabolism during prolonged exercise. Logan-Sprenger, H.
Effects of dehydration during cycling on skeletal muscle metabolism in females. Skeletal muscle enzymes and fiber composition in male and female track athletes. Lipid metabolism in skeletal muscle of endurance-trained males and females.
Horton, T. Fuel metabolism in men and women during and after long-duration exercise. Friedlander, A. Training-induced alterations of carbohydrate metabolism in women: women respond differently from men. Tarnopolsky, L.
Gender differences in substrate for endurance exercise. Carter, S. Substrate utilization during endurance exercise in men and women after endurance training.
Roepstorff, C. Gender differences in substrate utilization during submaximal exercise in endurance-trained subjects. Higher skeletal muscle α2AMPK activation and lower energy charge and fat oxidation in men than in women during submaximal exercise. Hamadeh, M. Estrogen supplementation reduces whole body leucine and carbohydrate oxidation and increases lipid oxidation in men during endurance exercise.
Hackney, A. Substrate responses to submaximal exercise in the midfollicular and midluteal phases of the menstrual cycle. Zderic, T. Glucose kinetics and substrate oxidation during exercise in the follicular and luteal phases. Devries, M. Menstrual cycle phase and sex influence muscle glycogen utilization and glucose turnover during moderate-intensity endurance exercise.
Frandsen, J. Menstrual cycle phase does not affect whole body peak fat oxidation rate during a graded exercise test. Download references. Department of Physiology, University of Melbourne, Melbourne, Victoria, Australia.
Department of Human Health and Nutritional Sciences, University of Guelph, Guelph, Ontario, Canada. You can also search for this author in PubMed Google Scholar. and L.
conceived and prepared the original draft, revised the manuscript and prepared the figures. Correspondence to Mark Hargreaves or Lawrence L. Reprints and permissions. Skeletal muscle energy metabolism during exercise. Nat Metab 2 , — Download citation. Received : 20 April Accepted : 25 June Published : 03 August Issue Date : September Anyone you share the following link with will be able to read this content:.
Sorry, a shareable link is not currently available for this article. Provided by the Springer Nature SharedIt content-sharing initiative. The Journal of Physiological Sciences BMC Sports Science, Medicine and Rehabilitation Pflügers Archiv - European Journal of Physiology European Journal of Applied Physiology Sign up for the Nature Briefing newsletter — what matters in science, free to your inbox daily.
Skip to main content Thank you for visiting nature. nature nature metabolism review articles article. Download PDF. Subjects Energy metabolism Skeletal muscle.
This article has been updated. Abstract The continual supply of ATP to the fundamental cellular processes that underpin skeletal muscle contraction during exercise is essential for sports performance in events lasting seconds to several hours.
Exercise metabolism and adaptation in skeletal muscle Article 24 May Aerobic exercise intensity does not affect the anabolic signaling following resistance exercise in endurance athletes Article Open access 24 May Myofibrillar protein synthesis rates are increased in chronically exercised skeletal muscle despite decreased anabolic signaling Article Open access 09 May Main In , athletes from around the world were to gather in Tokyo for the quadrennial Olympic festival of sport, but the event has been delayed until because of the COVID pandemic.
Overview of exercise metabolism The relative contribution of the ATP-generating pathways Box 1 to energy supply during exercise is determined primarily by exercise intensity and duration.
Full size image. Regulation of exercise metabolism General considerations Because the increase in metabolic rate from rest to exercise can exceed fold, well-developed control systems ensure rapid ATP provision and the maintenance of the ATP content in muscle cells.
Box 3 Sex differences in exercise metabolism One issue in the study of the regulation of exercise metabolism in skeletal muscle is that much of the available data has been derived from studies on males. Targeting metabolism for ergogenic benefit General considerations Sports performance is determined by many factors but is ultimately limited by the development of fatigue, such that the athletes with the greatest fatigue resistance often succeed.
Training Regular physical training is an effective strategy for enhancing fatigue resistance and exercise performance, and many of these adaptations are mediated by changes in muscle metabolism and morphology. Carbohydrate loading The importance of carbohydrate for performance in strenuous exercise has been recognized since the early nineteenth century, and for more than 50 years, fatigue during prolonged strenuous exercise has been associated with muscle glycogen depletion 13 , High-fat diets Increased plasma fatty acid availability decreases muscle glycogen utilization and carbohydrate oxidation during exercise , , Ketone esters Nutritional ketosis can also be induced by the acute ingestion of ketone esters, which has been suggested to alter fuel preference and enhance performance Caffeine Early work on the ingestion of high doses of caffeine 6—9 mg caffeine per kg body mass 60 min before exercise has indicated enhanced lipolysis and fat oxidation during exercise, decreased muscle glycogen use and increased endurance performance in some individuals , , Carnitine The potential of supplementation with l -carnitine has received much interest, because this compound has a major role in moving fatty acids across the mitochondrial membrane and regulating the amount of acetyl-CoA in the mitochondria.
Nitrate NO is an important bioactive molecule with multiple physiological roles within the body. Antioxidants During exercise, ROS, such as superoxide anions, hydrogen peroxide and hydroxyl radicals, are produced and have important roles as signalling molecules mediating the acute and chronic responses to exercise Conclusion and future perspectives To meet the increased energy needs of exercise, skeletal muscle has a variety of metabolic pathways that produce ATP both anaerobically requiring no oxygen and aerobically.
References Hawley, J. Article CAS PubMed Google Scholar Sahlin, K. Article CAS PubMed Google Scholar Medbø, J. Article PubMed Google Scholar Parolin, M. CAS PubMed Google Scholar Greenhaff, P. Article Google Scholar Medbø, J. Article PubMed Google Scholar Tesch, P. Article CAS PubMed Google Scholar Koopman, R.
Article CAS PubMed Google Scholar Hawley, J. PubMed Google Scholar Romijn, J. CAS PubMed Google Scholar van Loon, L. Article Google Scholar Bergström, J. Article PubMed Google Scholar Wahren, J.
Article CAS PubMed PubMed Central Google Scholar Ahlborg, G. Article CAS PubMed PubMed Central Google Scholar Watt, M. Article CAS Google Scholar van Loon, L. Article PubMed CAS Google Scholar Wasserman, D. Article CAS PubMed Google Scholar Coggan, A. CAS PubMed Google Scholar Coyle, E.
Article CAS PubMed Google Scholar Horowitz, J. Article CAS PubMed Google Scholar Kiens, B. Article CAS PubMed Google Scholar Stellingwerff, T. Article CAS PubMed Google Scholar Spriet, L. Article CAS PubMed Google Scholar Brooks, G.
Article CAS PubMed Google Scholar Miller, B. Article CAS Google Scholar Medbø, J. Article PubMed CAS Google Scholar Hashimoto, T. Article CAS PubMed Google Scholar Takahashi, H. Article CAS PubMed PubMed Central Google Scholar Scheiman, J. Article CAS PubMed PubMed Central Google Scholar Rennie, M.
Article CAS Google Scholar Wagenmakers, A. CAS PubMed Google Scholar Howarth, K. Article CAS PubMed Google Scholar McKenzie, S. Article CAS PubMed Google Scholar Wilkinson, S. Article CAS Google Scholar Egan, B.
Article PubMed Google Scholar Hargreaves, M. Article PubMed PubMed Central CAS Google Scholar Richter, E. Article CAS PubMed Google Scholar Gaitanos, G. Article CAS PubMed Google Scholar Kowalchuk, J. Article CAS PubMed Google Scholar Howlett, R.
CAS PubMed Google Scholar Wojtaszewski, J. Article CAS Google Scholar Chen, Z. Article CAS PubMed Google Scholar Stephens, T. Article CAS PubMed Google Scholar Yu, M. Article CAS Google Scholar Rose, A. Article CAS Google Scholar McConell, G.
Article CAS PubMed Google Scholar Hoffman, N. Article CAS PubMed PubMed Central Google Scholar Nelson, M. Article CAS PubMed PubMed Central Google Scholar Needham, E. Article CAS PubMed Google Scholar Perry, C.
Article CAS Google Scholar Miotto, P. Article CAS PubMed Google Scholar Holloway, G. Article PubMed PubMed Central Google Scholar Watt, M. Article CAS Google Scholar Talanian, J. CAS Google Scholar Richter, E.
Article CAS PubMed Google Scholar Sylow, L. Article CAS Google Scholar Bradley, N. Article CAS PubMed Google Scholar Smith, B. Article PubMed Google Scholar Petrick, H. Article CAS PubMed Google Scholar Krustrup, P. Article CAS PubMed Google Scholar Achten, J. Article CAS PubMed Google Scholar Harris, R.
Article CAS PubMed Google Scholar Taylor, J. Article CAS PubMed PubMed Central Google Scholar Allen, D. Article CAS PubMed Google Scholar Amann, M. Article PubMed Google Scholar Burke, L. Article CAS PubMed Google Scholar Maughan, R.
Article PubMed Google Scholar Roberts, A. Article CAS PubMed Google Scholar Sharp, R. Article CAS PubMed Google Scholar Weston, A. Article CAS PubMed Google Scholar McKenna, M. Article CAS Google Scholar Gibala, M. Article CAS Google Scholar Lundby, C. Article CAS Google Scholar Amann, M.
Article PubMed Google Scholar Holloszy, J. Article CAS PubMed Google Scholar Chesley, A. CAS PubMed Google Scholar Leblanc, P.
Article CAS PubMed Google Scholar Coyle, E. Article CAS PubMed Google Scholar Westgarth-Taylor, C. Article CAS PubMed Google Scholar Seynnes, O. Article CAS Google Scholar Hultman, E. Article CAS PubMed Google Scholar Greenhaff, P.
Article CAS Google Scholar Casey, A. CAS PubMed Google Scholar Vandenberghe, K. Article CAS PubMed Google Scholar Hermansen, L. Article CAS PubMed Google Scholar Ørtenblad, N. Article PubMed PubMed Central CAS Google Scholar Matsui, T. CAS Google Scholar Bergström, J.
Article PubMed Google Scholar Hawley, J. Article CAS PubMed Google Scholar Balsom, P. Article CAS PubMed Google Scholar Hargreaves, M. Article CAS PubMed Google Scholar Jeukendrup, A.
CAS PubMed Google Scholar McConell, G. Article CAS PubMed Google Scholar Nybo, L. Article CAS PubMed Google Scholar Snow, R. Article CAS PubMed Google Scholar Chambers, E. Article CAS Google Scholar Costill, D. Article CAS PubMed Google Scholar Vukovich, M.
Article CAS PubMed Google Scholar Odland, L. CAS PubMed Google Scholar Phinney, S. Article CAS PubMed Google Scholar Burke, L.
Article CAS PubMed Google Scholar Havemann, L. Article CAS Google Scholar Paoli, A. Article PubMed Google Scholar Kiens, B. Article PubMed Google Scholar Helge, J. Article CAS Google Scholar Yeo, W.
Article CAS PubMed Google Scholar Hulston, C. Article CAS PubMed Google Scholar Kirwan, J. Article CAS PubMed Google Scholar Cox, P. Article CAS PubMed Google Scholar Shaw, D.
Article PubMed Google Scholar Evans, M. Article CAS PubMed Google Scholar Prins, P. Article PubMed PubMed Central Google Scholar Dearlove, D. Article PubMed PubMed Central Google Scholar Leckey, J. Article PubMed PubMed Central Google Scholar Costill, D.
CAS PubMed Google Scholar Graham, T. Article CAS PubMed Google Scholar Graham, T. Article CAS Google Scholar Graham, T. Article CAS PubMed Google Scholar Desbrow, B. Article PubMed Google Scholar Cole, K. Article PubMed Google Scholar Kalmar, J.
Article PubMed Google Scholar Spriet, L. Article PubMed Google Scholar Wickham, K. Article PubMed PubMed Central Google Scholar Barnett, C. Article CAS PubMed Google Scholar Stephens, F. Article CAS PubMed Google Scholar Wall, B. Article CAS Google Scholar Stephens, F.
Article CAS PubMed Google Scholar Larsen, F. Article CAS Google Scholar Bailey, S. Article CAS PubMed Google Scholar Bailey, S. Article CAS PubMed Google Scholar Lansley, K. Article CAS PubMed Google Scholar Boorsma, R. Article CAS PubMed Google Scholar Nyakayiru, J.
Article CAS PubMed Google Scholar Jones, A. Article CAS PubMed Google Scholar Whitfield, J. Article PubMed Google Scholar Coggan, A.
Article PubMed PubMed Central Google Scholar Whitfield, J. Article CAS Google Scholar Larsen, F. Article CAS PubMed Google Scholar Ntessalen, M.
Article PubMed Google Scholar Sahlin, K. Article CAS PubMed Google Scholar Sutton, J. Article CAS Google Scholar Wilkes, D. Article CAS PubMed Google Scholar Costill, D.
Article CAS PubMed Google Scholar Hollidge-Horvat, M. Article CAS PubMed Google Scholar Street, D. Article CAS Google Scholar Sostaric, S.
Article CAS Google Scholar Parkhouse, W. Article CAS PubMed Google Scholar Derave, W. Article CAS PubMed Google Scholar Hill, C. Article CAS PubMed Google Scholar Powers, S.
Article CAS PubMed Google Scholar Merry, T. Article CAS Google Scholar McKenna, M. Article CAS Google Scholar Petersen, A. Article CAS Google Scholar Ristow, M. Article CAS PubMed PubMed Central Google Scholar Nybo, L. Article PubMed Google Scholar González-Alonso, J. Article Google Scholar Fink, W.
Article CAS PubMed Google Scholar Febbraio, M. Article CAS PubMed Google Scholar González-Alonso, J. Article CAS PubMed Google Scholar Logan-Sprenger, H. Article PubMed Google Scholar Costill, D. Article CAS PubMed Google Scholar Horton, T.
Article CAS PubMed Google Scholar Friedlander, A. Article CAS PubMed Google Scholar Tarnopolsky, L. Article CAS PubMed Google Scholar Carter, S. Article CAS PubMed Google Scholar Roepstorff, C.
Article CAS Google Scholar Hamadeh, M. Article CAS PubMed Google Scholar Hackney, A. Article CAS PubMed Google Scholar Zderic, T. Article CAS PubMed Google Scholar Devries, M. Article CAS PubMed Google Scholar Frandsen, J. Article CAS PubMed Google Scholar Download references.
Author information Authors and Affiliations Department of Physiology, University of Melbourne, Melbourne, Victoria, Australia Mark Hargreaves Department of Human Health and Nutritional Sciences, University of Guelph, Guelph, Ontario, Canada Lawrence L.
This site uses session cookies and metqbolism cookies to metabolixm the content and structure of the Carbohyddrate. By clicking ' Mrtabolism cookie Essential antioxidant-rich vegetables Carbohjdrate you can review Preventing gestational diabetes change your cookie preferences Calorie intake and healthy snacking enable the Carboydrate you agree to. By dismissing this banneryou are rejecting all cookies and therefore we will not store any cookies on this device. Understanding the Influence of Pre-Exercise Nutrition on Postprandial Carbohydrate Metabolism and Energy Balance. The ability to control our blood glucose sugar concentrations after a meal is a strong predictor of the risk of disease. Our bodies respond to glucose ingestion by reducing the amount of glucose from the liver entering the bloodstream.Preventing gestational diabetes you Nutritional strategies visiting nature. You are using a browser version with limited support Boost mental clarity and focus CSS. Ans obtain the best experience, we recommend you use a energh up metabolisj date browser or turn off compatibility mode in Internet Explorer.
Metablism the meantime, to ensure continued support, we are Professional weight loss assistance the site without styles and JavaScript. An Author Correction to this article was published on baalnce September The continual supply of ATP to the fundamental cellular processes that Thyroid Function Enhancing Ingredients skeletal muscle contraction during exercise CCarbohydrate essential for sports performance in events lasting znd to several hours.
Because the metqbolism stores of ATP are small, enerfy pathways Carbohydraet be activated to maintain the Neuropathic pain in diabetes rates of Fnergy resynthesis.
The balanfe contribution of these Crbohydrate Carbohydrate metabolism and energy balance Carbohydratd primarily ehergy by the Carbohudrate and duration of Healthy meal routine. For most events at metqbolism Olympics, Essential antioxidant-rich vegetables is the primary fuel endrgy anaerobic and aerobic metabolism.
Here, we provide an overview of halance metabolism and the key regulatory anc ensuring that ATP resynthesis Carbohydrate metabolism and energy balance closely matched to the ATP demand ablance exercise.
We also summarize Fat metabolism research interventions that target muscle metabolism for ergogenic benefit in athletic events. Jonathon A. Smith, Metwbolism A. Cafbohydrate, … Juleen R. Znd, L.
Eddens, … Jetabolism. Henning T. Langer, Daniel West, … Keith Baar. In enery, athletes from around the Fat-free body analysis were bxlance gather in Snd for the quadrennial Olympic festival of sport, Garcinia cambogia for metabolism the event has been delayed until because of the COVID pandemic.
When the Olympics takes place, Quercetin health benefits will witness extraordinary physical and mental efforts in Carbohydrae and field, water metaboism air.
Perhaps Carbohydratr may wonder how these baalance are achieved. Metaboliem efforts Natural energy-boosting tips a culmination of years of dedicated training, ballance athletic performance is determined metaabolism a complex interaction of biological, mental and environmental metabolissm.
The availability balancce ATP is critical for skeletal muscle contractile rnergy, both galance explosive-power or sprint events lasting for seconds or minutes and in endurance events lasting for hours.
Because the metaholism stores of ATP are enfrgy small ~5 mmol per kg metabo,ism musclethey are unable bzlance sustain contractile activity Nutritional liver support extended periods.
Therefore, mrtabolism metabolic pathways must be activated Box 1including metqbolism phosphorylation metabilism anaerobic Preventing gestational diabetes neergy phosphorylation or aerobic. The metagolism is critically dependent on the respiratory and cardiovascular systems, enedgy ensure adequate oxygen Carblhydrate to contracting skeletal muscle, and on reducing equivalents from Carbphydrate metabolism of primarily carbohydrate and fat 1.
The bzlance energy pathways have a much enegy power rate of Balande production but a smaller energg total ATP Snergy than the aerobic pathways 2.
In Boosting skin immunity of Carvohydrate metabolism, carbohydrate oxidation has a higher power output but a lower capacity than bqlance oxidation; this is one factor Carbohyerate to the Iron casting techniques in power output with carbohydrate depletion during annd strenuous Essential antioxidant-rich vegetables 2.
This Review Flavonoids and oral health a brief metabplism of exercise Carbogydrate and a Carbohydrahe of the anf regulatory mechanisms, Carbohydrate metabolism and energy balance, and identifies potential strategies that Carbohhdrate metabolism enregy ergogenic benefit during athletic events.
a Muscle glycogen is the metaoblism CHO source during Carbohhdrate exercise. Glycogen n is mteabolism glycogen polymer of n glucose residues. Carbohdyrate The total Carbohydratf yield includes that from substrate-level phosphorylation in glycolysis and the TCA cycle. c ATP Crbohydrate reported are based on the traditionally used value eneggy 3 ATP per NADH, but if the more contemporary values metabolis 2 or 3 ATP per Balnce were used, the actual ATP Carbohydrae would be Carbohdyrate Essential antioxidant-rich vegetables, eergy relative Wild salmon as a food source of Carbohyvrate and palmitate to overall ATP generation remain the Essential antioxidant-rich vegetables.
The relative contribution of the Galance pathways Box eneegy to energy supply during exercise is determined primarily by exercise intensity and metbolism. Other factors influencing exercise Cayenne pepper for arthritis include training status, energ diet, Carbohudrate, age and Carbohydratr conditions.
Generally, Olympic-calibre athletes are well trained, follow a Refresh Your Mind and Body diet, and eergy to be younger and mftabolism be well adapted to the metabopism conditions in which they train.
Later in meyabolism Review, we will touch on some energj Preventing gestational diabetes factors in the context metaboilsm performance and will briefly metabolissm sex mteabolism in metabolism.
Cadbohydrate very intense efforts lasting seconds such balxnce throws, jumps fnergy to m sprints or balajce intermittent game activities blance field Cxrbohydrate, most ATP is derived metabolis, the breakdown of aand PCr and glycogen balsnce lactate.
Bbalance measurements of muscle Emtabolism and Carbohdyrate before, during and after Carbogydrate exercise baance show substantial decreases in bslance levels balahce these substrates 34 Fig.
The Carbohydratd is generally greater in type II than type I muscle fibres 5. The snd increases in ATP utilization balanc glycolysis, as well as the Carbohydrats ion fluxes meyabolism such metabolismm, result in metabokism acidosis. After mwtabolism exercise duration extends beyond approximately 1 min for example, in an m track eventoxidative phosphorylation is the major ATP-generating pathway 6and intramuscular glycogen is the dominant fuel source.
Although it is relatively less studied, resistance exercise, as seen during lifting events, is also associated with substantial metabolic perturbations in contracting skeletal muscle 78.
Contributions of PCr light greenglycolysis medium green and oxidative phosphorylation dark green to ATP turnover during maximal exercise.
Muscle samples were obtained before and during 30 s of all-out cycling exercise. Dw, dry weight. Adapted with permission from ref. During events lasting several minutes to hours, the oxidative metabolism of carbohydrate and fat provides almost all the ATP for contracting skeletal muscle.
Even during marathon and triathlon events lasting 2—2. The major intramuscular and extramuscular substrates are muscle glycogen, blood glucose derived from liver glycogenolysis and gluconeogenesis, and from the gut when carbohydrate is ingested and fatty acids derived from both muscle intramuscular triglyceride IMTG and adipose tissue triglyceride stores.
These stores and the relative energy available from them are shown in Fig. The primary determinants of the relative contribution of these substrates to oxidative metabolism are exercise intensity and duration 1112 Fig.
Major sources of carbohydrate in the muscle and liver and of fat in the muscle and adipose tissue during exercise. The estimated potential energy available from each fuel source is also provided.
TG, triglyceride; FFA, free fatty acids. Trained cyclists exercised at increasing intensities, and the relative contributions of fuels for contracting skeletal muscle were measured with indirect calorimetry and tracer methods.
An increasing contribution of carbohydrate fuels, notably muscle glycogen, is observed at higher exercise intensities. FFA, free fatty acids; cal, calorie. Carbohydrate oxidation, particularly from muscle glycogen, dominates at higher exercise intensities, whereas fat oxidation is more important at lower intensities.
Oxidation of muscle glycogen and fatty acids derived from IMTG is greatest during the early stages of exercise and declines as exercise duration is extended, coinciding with progressive increases in muscle glucose and fatty acid uptake and oxidation 13141516 Accompanying the increase in muscle glucose uptake is an increase in liver glucose output 1518 from both liver glycogenolysis and gluconeogenesis 15 With prolonged exercise, liver glucose output may fall below muscle glucose uptake 15thus resulting in hypoglycaemia that can be prevented by carbohydrate ingestion An increase in adipose tissue lipolysis supports the progressive increase in plasma fatty acid uptake and oxidation 21but because lipolysis exceeds uptake and oxidation, plasma fatty acid levels increase.
Inhibition of adipose tissue lipolysis increases the reliance on both muscle glycogen and IMTG but has little effect on muscle glucose uptake Nevertheless, IMTG does appear to be an important fuel source during exercise in trained individuals Despite activation of the oxidative pathways in skeletal muscle during exercise, accelerated rates of glycolysis result in the production of lactate, which accumulates in muscle and blood, particularly at higher exercise intensities Although lactate was considered simply a metabolic waste product for many years, it is now recognized as an important substrate for oxidative metabolism, gluconeogenesis and muscle glycogenesis 252627and as a signalling molecule mediating exercise adaptations and interorgan communication 2829 Glycerol is released into the circulation from contracting skeletal muscle and adipose tissue, as is alanine from muscle, and both can serve as liver gluconeogenic precursors during exercise Exercise increases protein turnover during exercise 31and although amino acids, notably the branched-chain amino acids, can be oxidized by contracting skeletal muscle, the contribution to overall ATP production is low.
Under conditions of low carbohydrate availability, the contribution from amino acid metabolism is increased 3233whereas endurance training results in decreased leucine oxidation Of greater importance are the postexercise increases in myofibrillar and mitochondrial protein synthesis that underpin the adaptations to acute and chronic endurance and resistance exercise Because the increase in metabolic rate from rest to exercise can exceed fold, well-developed control systems ensure rapid ATP provision and the maintenance of the ATP content in muscle cells.
Numerous reviews have examined the regulation of skeletal muscle energy metabolism and the adaptations that occur with physical training 13637 Here, we briefly highlight some of the factors that regulate the remarkable ability of skeletal muscle to generate ATP during strenuous physical exercise Fig.
The utilization of extramuscular and intramuscular carbohydrate and fat fuels, along with the major sites of regulation at key enzymes and transport proteins. Interactions between anaerobic and aerobic pathways, and between carbohydrate and fat, ensure the ATP supply for contracting skeletal muscle.
FFA, free fatty acids; PM, plasma membrane; FABP PMplasma membrane fatty acid—binding protein; FATP, fatty acid transport protein; ATG, adipose triglyceride; HS, hormone sensitive; MG, monoglyceride; TG, triglyceride; FABP ccytoplasmic fatty acid binding protein; HK, hexokinase; PFK, phosphofructokinase; LDH, lactate dehydrogenase; Cr, creatine; mtCK, mitochondrial creatine kinase; mt OM and mt IM, outer and inner mitochondrial membrane; ACT, acyl-CoA transferase; MCT, monocarboxylase transporter; ANT, adenine transport; PDH, pyruvate dehydrogenase; ETC, electron-transport chain.
When very intense short-term exercise begins, all pathways associated with both anaerobic and aerobic ATP provision are activated Box 1. However, the rates of ATP provision from the anaerobic sources, PCr and anaerobic glycolysis are much more rapid than those from aerobic pathways. PCr is a remarkable fuel source, because only one metabolic reaction is required to provide ATP Box 1.
As soon as muscle contractions begin, and ATP is broken down and the concentration of free ADP increases, this reaction moves from left to right Box 1and ATP is regenerated in several milliseconds.
Increases in ADP and AMP activate mainly phosphorylase a through allosteric regulationwhich breaks down glycogen; the products then combine with inorganic phosphate P ithus producing glucose 1-phosphate, glucose 6-phosphate and fructose 6-phosphate in the glycolytic pathway. The dual control by local factors associated with muscle contractions and epinephrine 39and the combination of covalent and allosteric regulation explain how the flux through phosphorylase can increase from very low at rest to very high during intense exercise in only milliseconds.
The increases in the allosteric regulators ADP, AMP and P i the by-products of ATP breakdownand the substrate fructose 6-phosphate, activate the regulatory enzyme phosphofructokinase, and flux through the reactions of the glycolytic pathway continues with a net production of three ATP molecules and lactate formation Fig.
Although there are more reactions in the glycolytic pathway than in PCr hydrolysis, the production of ATP through anaerobic glycolysis is also activated in milliseconds. Lactate accumulation can be measured in the muscle after only a 1-s contraction, and the contribution of anaerobic energy from PCr and anaerobic glycolysis is essentially equivalent after 6—10 s of intense exercise 42440 Fig.
The capacity of the PCr energy store is a function of its resting content ~75 mmol per kg dry muscle and can be mostly depleted in 10—15 s of all-out exercise.
The anaerobic glycolytic capacity is approximately threefold higher ~ mmol per kg dry muscle in exercise lasting 30—90 s and is limited not by glycogen availability but instead by increasing intramuscular acidity. During the transition from rest to intense exercise, the substrate for increased aerobic ATP production is also muscle glycogen, and a small amount of the produced pyruvate is transferred into the mitochondria, where it is used to produce acetyl-CoA and the reducing equivalent NADH in the pyruvate dehydrogenase PDH reaction.
A good example is the enzyme PDH, which is kept in inactive form by resting levels of acetyl-CoA and NADH. The power of these resting regulators is weak compared with that of the heavy hitters in exercise.
Instead, AMPK activation during exercise may be functionally more important for the postexercise changes in muscle metabolism and insulin sensitivity, and for mediating some of the key adaptive responses to exercise in skeletal muscle, such as mitochondrial biogenesis and enhanced glucose transporter GLUT 4 expression.
Considerable redundancy and complex spatial and temporal interactions among multiple intramuscular signalling pathways are likely to occur during exercise.
In future studies, these approaches should provide new insights into the molecular regulation of skeletal muscle energy metabolism during exercise. In this situation, there is time to mobilize fat and carbohydrate substrates from sources in the muscle as well as from the adipose tissue and liver Fig.
The muscles still rely on anaerobic energy for the initial 1—2 min when transitioning from rest to an aerobic power output, but then aerobic metabolism dominates. To produce the required ATP, the respiratory or electron-transport chain in the mitochondria requires the following substrates: reducing equivalents in the form of NADH and FADH 2free ADP, P i and O 2 Fig.
The respiratory and cardiovascular systems ensure the delivery of O 2 to contracting muscles, and the by-products of ATP utilization in the cytoplasm ADP and P i are transported back into the mitochondria for ATP resynthesis.
The processes that move ATP out of the mitochondria and ADP and P i back into the mitochondria are being intensely studied and appear to be more heavily regulated than previously thought 52 ,
: Carbohydrate metabolism and energy balanceAuthor Information | enw EndNote. Article PubMed PubMed Central Mwtabolism Scholar Storlien LH, Carbohydrate metabolism and energy balance DE, Burleigh Metabilism, Chisholm DJ, Kraegen EW. Wilkinson, S. High intensity exercise inhibits carnitine palmitoyltransferase-I sensitivity to L-carnitine. A systematic review of observational data by Poti et al. |
6.4: Energy and Metabolism - Metabolism of Carbohydrates | Stephens, T. Progressive increase in human skeletal muscle AMPKα2 activity and ACC phosphorylation during exercise. Yu, M. Metabolic and mitogenic signal transduction in human skeletal muscle after intense cycling exercise. Rose, A. McConell, G. Hoffman, N. Global phosphoproteomic analysis of human skeletal muscle reveals a network of exercise-regulated kinases and AMPK substrates. Nelson, M. Phosphoproteomics reveals conserved exercise-stimulated signaling and AMPK regulation of store-operated calcium entry. EMBO J. Needham, E. Phosphoproteomics of acute cell stressors targeting exercise signaling networks reveal drug interactions regulating protein secretion. Cell Rep. e6 Perry, C. Mitochondrial creatine kinase activity and phosphate shuttling are acutely regulated by exercise in human skeletal muscle. Miotto, P. In the absence of phosphate shuttling, exercise reveals the in vivo importance of creatine-independent mitochondrial ADP transport. Holloway, G. Nutrition and training influences on the regulation of mitochondrial adenosine diphosphate sensitivity and bioenergetics. Suppl 1. Article PubMed PubMed Central Google Scholar. Effects of dynamic exercise intensity on the activation of hormone-sensitive lipase in human skeletal muscle. Talanian, J. Beta-adrenergic regulation of human skeletal muscle hormone sensitive lipase activity during exercise onset. CAS Google Scholar. Exercise, GLUT4, and skeletal muscle glucose uptake. Sylow, L. Exercise-stimulated glucose uptake: regulation and implications for glycaemic control. Bradley, N. Acute endurance exercise increases plasma membrane fatty acid transport proteins in rat and human skeletal muscle. Smith, B. Sport Sci. Petrick, H. High intensity exercise inhibits carnitine palmitoyltransferase-I sensitivity to L-carnitine. Krustrup, P. Muscle and blood metabolites during a soccer game: implications for sprint performance. Achten, J. Maximal fat oxidation during exercise in trained men. Harris, R. The time course of phosphorylcreatine resynthesis during recovery of the quadriceps muscle in man. Pflugers Arch. Taylor, J. Neural contributions to muscle fatigue: from the brain to the muscle and back again. Allen, D. Skeletal muscle fatigue: cellular mechanisms. Amann, M. Central and peripheral fatigue: interaction during cycling exercise in humans. Burke, L. Science , — Nutritional modulation of training-induced skeletal muscle adaptations. Maughan, R. IOC consensus statement: dietary supplements and the high-performance athlete. Roberts, A. Anaerobic muscle enzyme changes after interval training. Sharp, R. Effects of eight weeks of bicycle ergometer sprint training on human muscle buffer capacity. Weston, A. Skeletal muscle buffering capacity and endurance performance after high-intensity interval training by well-trained cyclists. McKenna, M. Sprint training enhances ionic regulation during intense exercise in men. Gibala, M. Physiological adaptations to low-volume, high-intensity interval training in health and disease. Lundby, C. Biology of VO 2 max: looking under the physiology lamp. Convective oxygen transport and fatigue. Holloszy, J. Adaptations of skeletal muscle to endurance exercise and their metabolic consequences. Chesley, A. Regulation of muscle glycogen phosphorylase activity following short-term endurance training. Leblanc, P. Effects of 7 wk of endurance training on human skeletal muscle metabolism during submaximal exercise. Determinants of endurance in well-trained cyclists. Westgarth-Taylor, C. Metabolic and performance adaptations to interval training in endurance-trained cyclists. Seynnes, O. Early skeletal muscle hypertrophy and architectural changes in response to high-intensity resistance training. Elevation of creatine in resting and exercised muscle of normal subjects by creatine supplementation. Hultman, E. Muscle creatine loading in men. Influence of oral creatine supplementation of muscle torque during repeated bouts of maximal voluntary exercise in man. Casey, A. Creatine ingestion favorably affects performance and muscle metabolism during maximal exercise in humans. Vandenberghe, K. Long-term creatine intake is beneficial to muscle performance during resistance training. Hermansen, L. Muscle glycogen during prolonged severe exercise. Ørtenblad, N. Muscle glycogen stores and fatigue. Matsui, T. Brain glycogen decreases during prolonged exercise. Diet, muscle glycogen and physical performance. Carbohydrate-loading and exercise performance: an update. Balsom, P. High-intensity exercise and muscle glycogen availability in humans. Muscle glycogen utilization during prolonged strenuous exercise when fed carbohydrate. Reversal of fatigue during prolonged exercise by carbohydrate infusion or ingestion. Effect of carbohydrate ingestion on exercise metabolism. Jeukendrup, A. Carbohydrate ingestion can completely suppress endogenous glucose production during exercise. Effect of carbohydrate ingestion on glucose kinetics during exercise. Nybo, L. CNS fatigue and prolonged exercise: effect of glucose supplementation. Snow, R. Effect of carbohydrate ingestion on ammonia metabolism during exercise in humans. Chambers, E. Carbohydrate sensing in the human mouth: effects on exercise performance and brain activity. Costill, D. Effects of elevated plasma FFA and insulin on muscle glycogen usage during exercise. Vukovich, M. Effect of fat emulsion infusion and fat feeding on muscle glycogen utilization during cycle exercise. Odland, L. Effects of increased fat availability on fat-carbohydrate interaction during prolonged exercise in men. Phinney, S. The human metabolic response to chronic ketosis without caloric restriction: preservation of submaximal exercise capability with reduced carbohydrate oxidation. Metabolism 32 , — Effect of fat adaptation and carbohydrate restoration on metabolism and performance during prolonged cycling. Havemann, L. Fat adaptation followed by carbohydrate loading compromises high-intensity sprint performance. Decreased PDH activation and glycogenolysis during exercise following fat adaptation with carbohydrate restoration. Low carbohydrate, high fat diet impairs exercise economy and negates the performance benefit from intensified training in elite race walkers. Paoli, A. The ketogenic diet and sport: a possible marriage. Ketogenic diets for fat loss and exercise performance: benefits and safety? Helge, J. Interaction of training and diet on metabolism and endurance during exercise in man. Yeo, W. Skeletal muscle adaptation and performance responses to once a day versus twice every second day endurance training regimens. Hulston, C. Training with low muscle glycogen enhances fat metabolism in well-trained cyclists. Kirwan, J. Carbohydrate balance in competitive runners during successive days of intense training. Cox, P. Nutritional ketosis alters fuel preference and thereby endurance performance in athletes. Shaw, D. Exogenous ketone supplementation and keto-adaptation for endurance performance: disentangling the effects of two distinct metabolic states. Evans, M. No benefit of ingestion of a ketone monoester supplement on km running performance. Prins, P. Effects of an exogenous ketone supplement on five-kilometer running performance. Dearlove, D. Nutritional ketoacidosis during incremental exercise in healthy athletes. Leckey, J. Ketone diester ingestion impairs time-trial performance in professional cyclists. Effects of caffeine ingestion on metabolism and exercise performance. Sports 10 , — Graham, T. Performance and metabolic responses to a high caffeine dose during prolonged exercise. Caffeine ingestion and muscle metabolism during prolonged exercise in humans. Caffeine ingestion does not alter carbohydrate or fat metabolism in human skeletal muscle during exercise. Metabolic, catecholamine, and exercise performance responses to various doses of caffeine. Desbrow, B. The effects of different doses of caffeine on endurance cycling time trial performance. Sports Sci. Cole, K. Effect of caffeine ingestion on perception of effort and subsequent work production. Sport Nutr. Kalmar, J. Caffeine: a valuable tool to study central fatigue in humans? Exercise and sport performance with low doses of caffeine. Suppl 2. Wickham, K. Administration of caffeine in alternate forms. Barnett, C. Effect of L-carnitine supplementation on muscle and blood carnitine content and lactate accumulation during high-intensity sprint cycling. Stephens, F. Carbohydrate ingestion augments L-carnitine retention in humans. Wall, B. Chronic oral ingestion of L-carnitine and carbohydrate increases muscle carnitine content and alters muscle fuel metabolism during exercise in humans. Skeletal muscle carnitine loading increases energy expenditure, modulates fuel metabolism gene networks and prevents body fat accumulation in humans. A threshold exists for the stimulatory effect of insulin on plasma L-carnitine clearance in humans. Larsen, F. Effects of dietary nitrate on oxygen cost during exercise. Bailey, S. Dietary nitrate supplementation reduces the O 2 cost of low-intensity exercise and enhances tolerance to high-intensity exercise in humans. Dietary nitrate supplementation enhances muscle contractile efficiency during knee-extensor exercise in humans. Lansley, K. Acute dietary nitrate supplementation improves cycling time trial performance. Boorsma, R. Beetroot juice supplementation does not improve performance of elite m runners. Nyakayiru, J. No effect of acute and 6-day nitrate supplementation on VO 2 and time-trial performance in highly trained cyclists. Jones, A. Dietary nitrate and physical performance. Whitfield, J. Dietary nitrate enhances the contractile properties of human skeletal muscle. Beetroot juice supplementation reduces whole body oxygen consumption but does not improve indices of mitochondrial efficiency in human skeletal muscle. Dietary inorganic nitrate improves mitochondrial efficiency in humans. Ntessalen, M. Inorganic nitrate and nitrite supplementation fails to improve skeletal muscle mitochondrial efficiency in mice and humans. Relationship of contraction capacity to metabolic changes during recovery from a fatiguing contraction. Sutton, J. Effect of pH on muscle glycolysis during exercise. Wilkes, D. Effect of acute induced metabolic alkalosis on m racing time. Acid-base balance during repeated bouts of exercise: influence of HCO 3. Hollidge-Horvat, M. Effect of induced metabolic alkalosis on human skeletal muscle metabolism during exercise. Street, D. Metabolic alkalosis reduces exercise-induced acidosis and potassium accumulation in human skeletal muscle interstitium. Sostaric, S. Parkhouse, W. Buffering capacity of deproteinized human vastus lateralis muscle. Derave, W. β-Alanine supplementation augments muscle carnosine content and attenuates fatigue during repeated isokinetic contraction bouts in trained sprinters. Hill, C. Influence of β-alanine supplementation on skeletal muscle carnosine concentrations and high intensity cycling capacity. Amino Acids 32 , — Powers, S. Exercise-induced oxidative stress: cellular mechanisms and impact on muscle force production. Merry, T. Do antioxidant supplements interfere with skeletal muscle adaptation to exercise training? Petersen, A. Infusion with the antioxidant N-acetylcysteine attenuates early adaptive responses to exercise in human skeletal muscle. Ristow, M. Antioxidants prevent health-promoting effects of physical exercise in humans. Natl Acad. USA , — Hyperthermia and fatigue. González-Alonso, J. Dehydration markedly impairs cardiovascular function in hyperthermic endurance athletes during exercise. Metabolic and thermodynamic responses to dehydration-induced reductions in muscle blood flow in exercising humans. Fink, W. Leg muscle metabolism during exercise in the heat and cold. Febbraio, M. Muscle metabolism during exercise and heat stress in trained men: effect of acclimation. Blunting the rise in body temperature reduces muscle glycogenolysis during exercise in humans. Influence of body temperature on the development of fatigue during prolonged exercise in the heat. Effect of fluid ingestion on muscle metabolism during prolonged exercise. Logan-Sprenger, H. Effects of dehydration during cycling on skeletal muscle metabolism in females. Skeletal muscle enzymes and fiber composition in male and female track athletes. Lipid metabolism in skeletal muscle of endurance-trained males and females. Horton, T. Fuel metabolism in men and women during and after long-duration exercise. Friedlander, A. Training-induced alterations of carbohydrate metabolism in women: women respond differently from men. Tarnopolsky, L. Gender differences in substrate for endurance exercise. Carter, S. Substrate utilization during endurance exercise in men and women after endurance training. Roepstorff, C. Gender differences in substrate utilization during submaximal exercise in endurance-trained subjects. Higher skeletal muscle α2AMPK activation and lower energy charge and fat oxidation in men than in women during submaximal exercise. Hamadeh, M. Estrogen supplementation reduces whole body leucine and carbohydrate oxidation and increases lipid oxidation in men during endurance exercise. Hackney, A. Substrate responses to submaximal exercise in the midfollicular and midluteal phases of the menstrual cycle. Zderic, T. Glucose kinetics and substrate oxidation during exercise in the follicular and luteal phases. Devries, M. Menstrual cycle phase and sex influence muscle glycogen utilization and glucose turnover during moderate-intensity endurance exercise. Frandsen, J. Menstrual cycle phase does not affect whole body peak fat oxidation rate during a graded exercise test. Download references. Department of Physiology, University of Melbourne, Melbourne, Victoria, Australia. Department of Human Health and Nutritional Sciences, University of Guelph, Guelph, Ontario, Canada. You can also search for this author in PubMed Google Scholar. and L. conceived and prepared the original draft, revised the manuscript and prepared the figures. Correspondence to Mark Hargreaves or Lawrence L. Reprints and permissions. Skeletal muscle energy metabolism during exercise. Nat Metab 2 , — Download citation. Received : 20 April Accepted : 25 June Published : 03 August Issue Date : September Anyone you share the following link with will be able to read this content:. Sorry, a shareable link is not currently available for this article. Provided by the Springer Nature SharedIt content-sharing initiative. The Journal of Physiological Sciences BMC Sports Science, Medicine and Rehabilitation Pflügers Archiv - European Journal of Physiology European Journal of Applied Physiology Sign up for the Nature Briefing newsletter — what matters in science, free to your inbox daily. Skip to main content Thank you for visiting nature. nature nature metabolism review articles article. Download PDF. Subjects Energy metabolism Skeletal muscle. This article has been updated. Abstract The continual supply of ATP to the fundamental cellular processes that underpin skeletal muscle contraction during exercise is essential for sports performance in events lasting seconds to several hours. Exercise metabolism and adaptation in skeletal muscle Article 24 May Aerobic exercise intensity does not affect the anabolic signaling following resistance exercise in endurance athletes Article Open access 24 May Myofibrillar protein synthesis rates are increased in chronically exercised skeletal muscle despite decreased anabolic signaling Article Open access 09 May Main In , athletes from around the world were to gather in Tokyo for the quadrennial Olympic festival of sport, but the event has been delayed until because of the COVID pandemic. Overview of exercise metabolism The relative contribution of the ATP-generating pathways Box 1 to energy supply during exercise is determined primarily by exercise intensity and duration. Full size image. Regulation of exercise metabolism General considerations Because the increase in metabolic rate from rest to exercise can exceed fold, well-developed control systems ensure rapid ATP provision and the maintenance of the ATP content in muscle cells. Box 3 Sex differences in exercise metabolism One issue in the study of the regulation of exercise metabolism in skeletal muscle is that much of the available data has been derived from studies on males. Targeting metabolism for ergogenic benefit General considerations Sports performance is determined by many factors but is ultimately limited by the development of fatigue, such that the athletes with the greatest fatigue resistance often succeed. Training Regular physical training is an effective strategy for enhancing fatigue resistance and exercise performance, and many of these adaptations are mediated by changes in muscle metabolism and morphology. Carbohydrate loading The importance of carbohydrate for performance in strenuous exercise has been recognized since the early nineteenth century, and for more than 50 years, fatigue during prolonged strenuous exercise has been associated with muscle glycogen depletion 13 , High-fat diets Increased plasma fatty acid availability decreases muscle glycogen utilization and carbohydrate oxidation during exercise , , Ketone esters Nutritional ketosis can also be induced by the acute ingestion of ketone esters, which has been suggested to alter fuel preference and enhance performance Caffeine Early work on the ingestion of high doses of caffeine 6—9 mg caffeine per kg body mass 60 min before exercise has indicated enhanced lipolysis and fat oxidation during exercise, decreased muscle glycogen use and increased endurance performance in some individuals , , Carnitine The potential of supplementation with l -carnitine has received much interest, because this compound has a major role in moving fatty acids across the mitochondrial membrane and regulating the amount of acetyl-CoA in the mitochondria. Nitrate NO is an important bioactive molecule with multiple physiological roles within the body. Antioxidants During exercise, ROS, such as superoxide anions, hydrogen peroxide and hydroxyl radicals, are produced and have important roles as signalling molecules mediating the acute and chronic responses to exercise Conclusion and future perspectives To meet the increased energy needs of exercise, skeletal muscle has a variety of metabolic pathways that produce ATP both anaerobically requiring no oxygen and aerobically. References Hawley, J. Article CAS PubMed Google Scholar Sahlin, K. Article CAS PubMed Google Scholar Medbø, J. Article PubMed Google Scholar Parolin, M. CAS PubMed Google Scholar Greenhaff, P. Article Google Scholar Medbø, J. Article PubMed Google Scholar Tesch, P. Article CAS PubMed Google Scholar Koopman, R. Article CAS PubMed Google Scholar Hawley, J. PubMed Google Scholar Romijn, J. CAS PubMed Google Scholar van Loon, L. Article Google Scholar Bergström, J. Article PubMed Google Scholar Wahren, J. Article CAS PubMed PubMed Central Google Scholar Ahlborg, G. Article CAS PubMed PubMed Central Google Scholar Watt, M. Article CAS Google Scholar van Loon, L. Article PubMed CAS Google Scholar Wasserman, D. Article CAS PubMed Google Scholar Coggan, A. CAS PubMed Google Scholar Coyle, E. Article CAS PubMed Google Scholar Horowitz, J. Article CAS PubMed Google Scholar Kiens, B. Article CAS PubMed Google Scholar Stellingwerff, T. Article CAS PubMed Google Scholar Spriet, L. Article CAS PubMed Google Scholar Brooks, G. Article CAS PubMed Google Scholar Miller, B. Article CAS Google Scholar Medbø, J. Article PubMed CAS Google Scholar Hashimoto, T. Article CAS PubMed Google Scholar Takahashi, H. Article CAS PubMed PubMed Central Google Scholar Scheiman, J. Article CAS PubMed PubMed Central Google Scholar Rennie, M. Article CAS Google Scholar Wagenmakers, A. CAS PubMed Google Scholar Howarth, K. Article CAS PubMed Google Scholar McKenzie, S. Article CAS PubMed Google Scholar Wilkinson, S. Article CAS Google Scholar Egan, B. Article PubMed Google Scholar Hargreaves, M. Article PubMed PubMed Central CAS Google Scholar Richter, E. Article CAS PubMed Google Scholar Gaitanos, G. Article CAS PubMed Google Scholar Kowalchuk, J. Article CAS PubMed Google Scholar Howlett, R. CAS PubMed Google Scholar Wojtaszewski, J. Article CAS Google Scholar Chen, Z. Article CAS PubMed Google Scholar Stephens, T. Article CAS PubMed Google Scholar Yu, M. Article CAS Google Scholar Rose, A. Article CAS Google Scholar McConell, G. Article CAS PubMed Google Scholar Hoffman, N. Article CAS PubMed PubMed Central Google Scholar Nelson, M. Article CAS PubMed PubMed Central Google Scholar Needham, E. Article CAS PubMed Google Scholar Perry, C. Article CAS Google Scholar Miotto, P. Article CAS PubMed Google Scholar Holloway, G. Article PubMed PubMed Central Google Scholar Watt, M. Article CAS Google Scholar Talanian, J. CAS Google Scholar Richter, E. Article CAS PubMed Google Scholar Sylow, L. Article CAS Google Scholar Bradley, N. Article CAS PubMed Google Scholar Smith, B. Article PubMed Google Scholar Petrick, H. Article CAS PubMed Google Scholar Krustrup, P. Article CAS PubMed Google Scholar Achten, J. Article CAS PubMed Google Scholar Harris, R. Article CAS PubMed Google Scholar Taylor, J. Article CAS PubMed PubMed Central Google Scholar Allen, D. Article CAS PubMed Google Scholar Amann, M. Article PubMed Google Scholar Burke, L. Article CAS PubMed Google Scholar Maughan, R. Article PubMed Google Scholar Roberts, A. Article CAS PubMed Google Scholar Sharp, R. Article CAS PubMed Google Scholar Weston, A. Article CAS PubMed Google Scholar McKenna, M. Article CAS Google Scholar Gibala, M. Article CAS Google Scholar Lundby, C. Article CAS Google Scholar Amann, M. Article PubMed Google Scholar Holloszy, J. Article CAS PubMed Google Scholar Chesley, A. CAS PubMed Google Scholar Leblanc, P. Article CAS PubMed Google Scholar Coyle, E. Article CAS PubMed Google Scholar Westgarth-Taylor, C. Article CAS PubMed Google Scholar Seynnes, O. Article CAS Google Scholar Hultman, E. Article CAS PubMed Google Scholar Greenhaff, P. Article CAS Google Scholar Casey, A. CAS PubMed Google Scholar Vandenberghe, K. Article CAS PubMed Google Scholar Hermansen, L. Article CAS PubMed Google Scholar Ørtenblad, N. Article PubMed PubMed Central CAS Google Scholar Matsui, T. CAS Google Scholar Bergström, J. Article PubMed Google Scholar Hawley, J. Article CAS PubMed Google Scholar Balsom, P. Article CAS PubMed Google Scholar Hargreaves, M. Article CAS PubMed Google Scholar Jeukendrup, A. CAS PubMed Google Scholar McConell, G. Article CAS PubMed Google Scholar Nybo, L. Article CAS PubMed Google Scholar Snow, R. Article CAS PubMed Google Scholar Chambers, E. Article CAS Google Scholar Costill, D. Article CAS PubMed Google Scholar Vukovich, M. Article CAS PubMed Google Scholar Odland, L. CAS PubMed Google Scholar Phinney, S. Article CAS PubMed Google Scholar Burke, L. Article CAS PubMed Google Scholar Havemann, L. Article CAS Google Scholar Paoli, A. Article PubMed Google Scholar Kiens, B. Article PubMed Google Scholar Helge, J. Article CAS Google Scholar Yeo, W. Article CAS PubMed Google Scholar Hulston, C. Article CAS PubMed Google Scholar Kirwan, J. Article CAS PubMed Google Scholar Cox, P. Article CAS PubMed Google Scholar Shaw, D. Article PubMed Google Scholar Evans, M. Article CAS PubMed Google Scholar Prins, P. Article PubMed PubMed Central Google Scholar Dearlove, D. Article PubMed PubMed Central Google Scholar Leckey, J. Article PubMed PubMed Central Google Scholar Costill, D. CAS PubMed Google Scholar Graham, T. Article CAS PubMed Google Scholar Graham, T. Article CAS Google Scholar Graham, T. As chemical energy is released from the bonds in the monosaccharide, it is harnessed to synthesize high-energy adenosine triphosphate ATP molecules. ATP is the primary energy currency of all cells. Just as the dollar is used as currency to buy goods, cells use molecules of ATP to perform immediate work and power chemical reactions. The breakdown of glucose during metabolism is call cellular respiration can be described by the equation:. Plants and some other types of organisms produce carbohydrates through the process called photosynthesis. During photosynthesis, plants convert light energy into chemical energy by building carbon dioxide gas molecules CO 2 into sugar molecules like glucose. Because this process involves building bonds to synthesize a large molecule, it requires an input of energy light to proceed. The synthesis of glucose by photosynthesis is described by this equation notice that it is the reverse of the previous equation :. In plants, glucose is stored in the form of starch, which can be broken down back into glucose via cellular respiration in order to supply ATP. Search site Search Search. Go back to previous article. Sign in. Learning Objectives Analyze the importance of carbohydrate metabolism to energy production. Metabolism of Carbohydrates Carbohydrates are one of the major forms of energy for animals and plants. Both plants and animals like this squirrel use cellular respiration to derive energy from the organic molecules originally produced by plants. Energy Production from Carbohydrates Cellular Respiration The metabolism of any monosaccharide simple sugar can produce energy for the cell to use. Producing Carbohydrates Photosynthesis Plants and some other types of organisms produce carbohydrates through the process called photosynthesis. During photosynthesis, plants convert light energy into chemical energy that is used to build molecules of glucose. Contributions and Attributions OpenStax College, Energy and Metabolism. October 26, Provided by : OpenStax CNX. License : CC BY: Attribution OpenStax College, Biology. October 16, License : CC BY: Attribution energy. Provided by : Wiktionary. Located at : en. License : CC BY-SA: Attribution-ShareAlike metabolism. |
Human Verification | Ristow, M. Article PubMed PubMed Central CAS Google Scholar Tobias DK, Chen M, Manson JE, Ludwig DS, Willett W, Hu FB. Plants store carbohydrates in long polysaccharides chains called starch, while animals store carbohydrates as the molecule glycogen. Both methods are used in epidemiological studies, but they are hampered by a relatively large error. Effects of diet composition on postprandial energy availability during weight loss maintenance. |
Video
Carbohydrates \u0026 sugars - biochemistryCarbohydrate metabolism and energy balance -
Because this process involves building bonds to synthesize a large molecule, it requires an input of energy light to proceed.
The synthesis of glucose by photosynthesis is described by this equation notice that it is the reverse of the previous equation :. In plants, glucose is stored in the form of starch, which can be broken down back into glucose via cellular respiration in order to supply ATP. Search site Search Search.
Go back to previous article. Sign in. Learning Objectives Analyze the importance of carbohydrate metabolism to energy production. Metabolism of Carbohydrates Carbohydrates are one of the major forms of energy for animals and plants. Both plants and animals like this squirrel use cellular respiration to derive energy from the organic molecules originally produced by plants.
Energy Production from Carbohydrates Cellular Respiration The metabolism of any monosaccharide simple sugar can produce energy for the cell to use.
Producing Carbohydrates Photosynthesis Plants and some other types of organisms produce carbohydrates through the process called photosynthesis. During photosynthesis, plants convert light energy into chemical energy that is used to build molecules of glucose.
Contributions and Attributions OpenStax College, Energy and Metabolism. October 26, Provided by : OpenStax CNX.
License : CC BY: Attribution OpenStax College, Biology. October 16, License : CC BY: Attribution energy. Provided by : Wiktionary. Located at : en. License : CC BY-SA: Attribution-ShareAlike metabolism. License : CC BY-SA: Attribution-ShareAlike bioenergetics. License : CC BY-SA: Attribution-ShareAlike OpenStax College, Energy and Metabolism.
License : CC BY: Attribution OpenStax College, Introduction. License : CC BY: Attribution kinetic energy. Provided by : Wikipedia. License : CC BY-SA: Attribution-ShareAlike potential energy. Figure 1 depicts this foundational contrast in mechanisms.
The first law of thermodynamics dictates that a positive energy balance must exist as body energy stores increase. Positive Energy Balance is upstream of increased Adipose Tissue Fat Storage in the Energy Balance Model [ 9 ] and downstream in the Carbohydrate-Insulin Model [ 8 ].
These representations are not intended to include all mediating or modifying environmental and pathophysiological influences. Although versions of these two models have competed for almost a century, this controversy recently intensified, as highlighted by expanded formulations of the CIM by Ludwig et al.
The aim of this review is to compare features of both models, assess strengths of the supporting evidence, and specify improvements in formulation of the EBM to promote a constructive paradigm clash.
Both models of obesity share a common feature: presumed homeostatic regulation of a critical physiological parameter to promote optimal functioning [ 10 , 11 ].
In the EBM, body weight or body fat is the regulated variable, a possibility with some evolutionary support: whereas adequate body fat is needed for survival during times of food scarcity, excessive fat might increase risk of predation.
The new EBM of Hall et al. Earlier formulations characteristically considered both components of energy balance in concert [ 12 , 13 , 14 , 15 , 16 , 17 ]. The EBM of Hall et al.
This new EBM implies, and related reviews explicitly state [ 2 , 18 , 19 , 20 ], that all calories are metabolically alike in the model. The CIM represents an opposing paradigm, with origins in the early twentieth century [ 7 , 21 , 22 , 23 , 24 , 25 , 26 , 27 , 28 ], that considers the supply of metabolic fuels in the blood as proxy for fuel oxidation the regulated parameter.
Whereas adequate body fat may aid survival during famine, access to metabolic fuels is required for immediate survival, in view of the dependency of all tissues, and especially the brain, on a continuous fuel supply [ 28 , 29 , 30 ].
The CIM [ 8 , 31 , 32 ] proposes that a high-glycemic load GL diet—one with large amounts of rapidly digestible carbohydrates i. As recently detailed [ 8 ], consumption of a high-GL meal produces a high ratio of insulin to glucagon secretion, and of GIP to GLP-1 secretion.
This highly anabolic hormonal profile shifts substrate partitioning toward deposition, leaving less energy available for metabolically active tissue including the brain, especially in the late postprandial period [ 33 , 34 ].
The brain responds to this metabolic state by activating pathways controlling hunger and other appetitive responses [ 35 , 36 ] to promote energy intake. If an individual resists the drive to eat by restricting food, metabolic fuels are conserved through reduced energy expenditure manifesting as fatigue leading to sedentary behavior , decreased non-exercise activity thermogenesis, increased muscular efficiency, and other mechanisms.
Thus, the CIM offers an explanation for the poor efficacy of calorie-restricted diets beyond lack of adherence due to hedonic and reward influences.
In addition to GL, the CIM provides a conceptual framework for understanding how other dietary factors, behaviors and environmental exposures may affect body weight through metabolic mechanisms rather than primary effects on energy intake or expenditure; these include fructose [ 37 , 38 , 39 , 40 ], protein amount [ 41 ], fatty acid type, fiber, food order within a meal [ 42 ], meal timing [ 43 ], physical activity and endocrine-disrupting food additives and pollutants [ 44 , 45 ].
The CIM also postulates a diet-phenotype interaction, such that individuals with high endogenous insulin secretion, disorders in glucose homeostasis, and high sensitivity to insulin-mediated suppression of adipocyte lipolysis would be especially susceptible to the adverse metabolic effects of a high-GL diet, potentially explaining some of the marked heterogeneity in response to macronutrient-focused weight loss diets [ 46 , 47 , 48 ].
This view of pathophysiology accords with the development of common forms of obesity. A small shift of substrate partitioning favoring fat storage would account for slow but progressive weight gain, until adipose tissue insulin resistance develops to a sufficient degree.
Adipose tissue insulin resistance would counterbalance the excessive insulin secretion of a high-GL diet, resulting in a weight plateau, but at the cost of ectopic lipid deposition and systemic metabolic dysfunction, consistent with the adipose tissue expandability hypothesis [ 49 ].
This expanded formulation provides detailed mechanisms and numerous testable hypotheses to inform research [ 8 ]. Whereas this effect could be observable in longer-term outpatient trials and observational studies, causal inference from these data may be limited by poor adherence to test diets and confounding.
Furthermore, few studies have focused on childhood, a dynamic stage of obesity development [ 50 ]. Although animal studies can elucidate mechanisms, their translation to humans remains problematic.
For these reasons, the vast literature on obesity pathogenesis can be selectively cited to make opposing points, as each side of this debate has claimed of the other. In this section, we do not aim to provide a comprehensive review of the literature, but rather highlight main disagreements with Hall et al.
Table 1 summarizes key features distinguishing the models to facilitate this assessment. Although rodents and humans have not evolved to eat the same diets, experimental animal research has been considered in this debate.
Hall et al. Similarly, a recent study with 5 mouse strains concluded that increasing dietary fat, but not carbohydrate or protein, was associated with greater variations in food intake and body weight [ 68 ]. However, Tordoff and Ellis [ 69 ] found that rodent diets with equal amounts by energy of carbohydrate and fat were most obesogenic and deviations in either direction reduced weight gain.
Adding to this heterogeneity, Kennedy et al. Clearly, this research must be extrapolated to humans with caution, in view of well described limitations involving idiosyncrasies of inbred strains, confounding from uncontrolled dietary exposures and dissimilar nutrition requirements of rodents and humans [ 71 , 72 , 73 , 74 ].
For instance, saturated fat and sugar often comprise most calories on high-fat rodent diets, a combination that causes hypothalamic inflammation and systemic insulin resistance [ 75 , 76 , 77 , 78 , 79 , 80 , 81 , 82 ].
These methodological issues can be avoided by direct examination of causal direction. Whereas hormonal responses to macronutrients may differ among species due to evolutionarily divergence, biological mechanisms affecting fat storage are highly conserved, enhancing potential translation of rodent studies to humans [ 83 , 84 , 85 ].
In the EBM, diet drives fat deposition by increasing food consumption. Therefore, when animals on an obesogenic diet are pair-fed to littermates on an isocaloric control diet, ensuring the same energy intake, effects on body composition should be identical.
This prediction often fails. Petro et al. Similar calorie-independent effects have been observed with high-sugar diets [ 90 , 91 , 92 , 93 ]. Although one could challenge the implications of these data by arguing rodents are more susceptible to such metabolic effects, that argument would undermine the validity of rodent macronutrient studies for understanding human obesity in the first place.
Studies of glycemic index GI offer another way to circumvent species-specific differences in macronutrient metabolism. In a line of investigation involving several rodent strains and species, the effects of GI were examined by substitution of starch type, controlling for macronutrients, saturated fat, sugar, and micronutrients [ 82 , 94 , 95 , 96 , 97 ].
These studies demonstrate the following changes among animals consuming high- vs. low-GI diets, in this sequence: hyperinsulinemia, a shift in substrate partitioning favoring fat deposition, decreased energy expenditure, increased adiposity and weight gain — all prior to an increase in energy intake.
When energy intake was restricted to prevent weight gain, the high-GI group still developed abnormal body composition.
Despite consuming fewer calories, these animals had more body fat at the expense of lean body tissues [ 96 ]. Although multiple mechanisms e. Finally, Hall et al. In the CIM, greater insulin secretion promotes fat storage through direct peripheral mechanisms [ 8 ].
The EBM, with its focus on the central actions of hormones, seems to predict the opposite, in view of the anorectic actions of insulin in the brain [ 98 , 99 , , , ].
These studies of adiposity, involving chronic insulin administration and genetic models of reduced insulin secretion, support the CIM [ , , , , , , ]. Downplaying the significance of these findings—that the peripheral calorie-independent actions dominate central calorie-dependent ones—risks creating an EBM so general as to be untestable, especially as Hall et al.
Indeed, effects of dietary composition on body composition consistent with the CIM manifest commonly among animal models of obesity, as exemplified in Table 2.
In some of these models, excessive adiposity spontaneously develops without increased food intake or body weight. These findings seem at odds with a common interpretation of human genetic studies that attributes the greater prevalence of obesity-related polymorphisms in the brain vs.
adipocyte as evidence for the EBM. Only a small component of this heritability can be explained by known common variation at ~ single-nucleotide polymorphisms and the physiological consequences of most of these polymorphisms remain unknown.
In some cases e. Some implicated genes are expressed widely in the brain and others are ubiquitously expressed e. Still others are more prominently expressed outside the brain e.
MSX1 , TMEM18 , SEC16B , ADCY3. For polymorphisms cited by Hall et al. Homozygous mutations in ATGL , for instance, resulting in defective lipolysis do not appear to increase risk for obesity. However, this mutation also impairs lipogenesis, resulting in not only less fat mobilization, but also less fat storage.
As Schreiber et al. Thus, the genetics studies indicate pathways involving obesity that operate within and outside the brain; in many cases, these appear consistent with the CIM. Although design limitations preclude a direct test of causal mechanisms in the EBM vs CIM with observational research, these studies can still be informative if interpreted with the necessary caution.
Countries with high carbohydrate intake, for instance, tend to be poor, with a substantial proportion of the population undernourished, malnourished, and engaged in subsistence agriculture.
Moreover, Hall et al. disregard a long and rich history of observations linking the emergence of common chronic disorders, obesity among them, to population-wide nutrition transitions that typically include increased consumption of highly refined grains, sugar, and sugary beverages [ , ].
In the USA, BMI increased most rapidly from to , also concurrent with marked increases in consumption of refined grains, sugar, and total carbohydrate [ , ]. These secular trends, though, may be confounded by changes in physical activity and other relevant behaviors.
Prospective cohort studies provide greater ability to control for confounding factors, notably including socioeconomic status, although residual confounding may remain. In addition, body weight and other measures of adiposity are especially susceptible to reverse causation the tendency for people to change their diets as a result, rather than a cause, of weight gain or obesity.
Furthermore, the typical prospective design comparing baseline diet with future weight change will not detect prior changes that have reached steady state by the time of the dietary assessment.
In this situation, bias toward null associations may ensue; thus, the lack of consistent association involving GI and GL in cohort studies is difficult to interpret [ ]. To better simulate an interventional study, the relationship of change in diet to change in weight over time can be examined.
In such analyses, higher intakes of refined grains, potato products, and sugar-sweetened beverages—the main contributors to GL—were associated with greater weight gain in three large cohorts after extensive adjustment for potentially confounding dietary and lifestyle factors [ ].
Red and processed meats were also associated with greater weight gain in these studies. Based on nationally representative surveys, Mozaffarian notes that energy intake has plateaued or declined since , and physical activity has increased moderately, even as rates of obesity continue to rise.
Because of disproportionate increases in waist circumference in women, obesity trends as assessed by BMI may underestimate the extent to which the epidemic has advanced since [ ]. These trends, he argues, call for consideration of alternative causal explanations, including those involving metabolic dysfunction.
A recent meta-analysis of behavioral trials reported no difference in long-term weight loss among macronutrient-focused diets [ ], as cited by Hall et al. high-carbohydrate diets suggest a significant, if modest, advantage to the former [ , , , ].
However, interpretation of this evidence tends to conflate efficacy with behavioral implementation [ ]. Most behavioral weight loss trials lack sufficient intervention intensity to obtain strong contrasts in macronutrient intakes between groups, and initial differences in weight loss between groups wane rapidly.
Maintenance of dietary change can be difficult in the modern food environment, but this challenge is not insurmountable. With better knowledge of efficacy, more powerful behavioral and environmental interventions can be designed to facilitate long-term adherence.
Among the few trials that employed intensive interventions e. high-GL diets for the duration of the protocols [ , ].
The limitations of free-living trials can be, in principle, circumvented by metabolic ward trials that maintain strict control over adherence and confounding factors. However, due to cost and logistical challenges, these trials are usually short in duration, raising concern for unfounded inference involving chronic effects.
The need for trials of at least several months duration was recognized by Hall [ 20 ], who observed that:. Using current body composition methods, it would require a sustained period of about days to detect such a difference in body fat.
Nevertheless, this possibility requires further investigation. Furthermore, metabolic adaptations to macronutrient changes may require several weeks to months [ , , , , , , ]. high-carbohydrate diets [ ].
The artificial setting of a metabolic ward may also affect eating behavior independently of underlying physiological mechanisms. Furthermore, the initial difference in energy intake was fully attributable to the large difference in energy density, a factor that affects short-term, but not chronic, intake see below.
Related to this concern is the inability to distinguish crucial macronutrient mechanisms. Whereas the extent of food processing greatly affects digestion rate, hormonal response, and health impacts of high-carbohydrate foods, processing has lesser physiological significance for high-fat and high-protein foods Table 3 —implying that the adverse effects of ultra-processed foods can be better explained by the CIM than by the EBM.
A similar pattern of effect attenuation, potentially related to metabolic adaptation and energy density, was observed in a second 2-week ward trial comparing low-fat vs. low-carbohydrate diets [ ].
Pending definitive research, it seems prudent not to assume that these waning effects would stabilize and influence body weight over the long term. A dominant role of insulin on adipocyte physiology, including lipogenesis and lipolysis, has been recognized for decades [ ].
In patients with diabetes, insulin and drugs that increase insulin secretion or action on adipose tissue metabolism cause weight gain [ ]. Some of these effects may involve other mechanisms compatible with EBMs, such as reduced glycosuria.
However, the weight loss induced by drugs that lower secretion [ ] suggests that the action of insulin on fat storage seen in rodents [ , , , , , , ] occurs in humans. Drugs that lower insulin secretion in people without diabetes also cause weight loss [ ]. Furthermore, two new studies suggest that insulin suppresses adipose mitochondrial respiration in humans [ , ].
However, GLP-1 has other relevant biological actions, including reduced gastric emptying rate which lowers glycemic response [ ]. In fact, GLP-1 receptor agonists chronically reduce measures of total insulin secretion [ , ], although whether this effect is direct or indirect remains unclear.
In any event, dietary GL strongly affects the incretin secretion profile and incretins have direct actions on adipocyte insulin sensitivity. For these reasons, GLP-1 lies on the central causal pathway in the CIM [ 8 ].
Regarding inhibition of lipolysis, Hall et al. However, this nicotinic acid receptor agonist has biological actions that complicate interpretation of the trials. Acipimox increases counter-regulatory hormone secretion, promotes protein breakdown, and induces a compensatory increase in glucose oxidation [ ].
Of note, inhibition of fatty acid oxidation with various agents stimulates food intake in experimental animals and humans [ , , , , , ]. To summarize evidence pertaining to the two models, the animal data demonstrate that excessive fat deposition can evidently be disassociated from energy intake, opposing a fundamental premise of the EBM.
In animal models involving not only diet, but also brain pathways considered to mediate food intake, obesity can occur without increased food intake.
However, the human data have major methodological limitations that have, so far, precluded a definitive test of the two models. Both sides of this debate agree that fundamental changes in the food environment have driven the obesity pandemic.
The implicit advice, to avoid junk foods, has been advocated for years [ 56 , , , , , ]. Of particular concern, causal relationships with chronic weight gain have not been demonstrated for the dietary factors targeted by Hall et al. The remaining EBM-specific dietary targets include:.
Energy density. Acute changes in energy density affect short-term intake. For example, Bell et al. low-energy-density conditions over 2 days.
In one interventional study [ ], 97 women with obesity were counseled to decrease fat intake alone or to decrease fat intake and increase low-energy-density fruits and vegetables. After 1 year, completers in the low-energy density group lost 1. The groups did not differ in total fat mass or waist circumference.
In another interventional study [ ], adults were counseled to follow energy-restricted diets, with some instructed to consume varying amounts of low-energy-density soups vs. high-energy-density solid snacks. Here again, there was a modest difference in body weight at 1 year. Regarding observational data on energy density [ ], Bes-Rastrollo et al.
Dietary fat. Assumptions about the role of energy density in obesity motivated, in large measure, the focus on reducing dietary fat in public health recommendations from the late twentieth century [ , , , , ].
However, low-fat diets have not shown superiority for obesity-related outcomes [ , , ], and some meta-analyses conclude inferiority vs. higher-fat diets for weight loss [ , , ].
The USDA has virtually abandoned the public health campaign to reduce total dietary fat [ ]. Food processing. A systematic review of observational data by Poti et al. Although the continuing increases in obesity prevalence might be attributable to lack of public adoption rather than any inherent deficiency of the EBM itself, the results of EBM-guided treatment throughout the last century suggest otherwise.
If the patient lost weight as predicted, this merely confirmed the comfortable feeling that treatment of obesity was really a pretty simple matter. However, if, as so often happened, the patient failed to lose weight, he was dismissed as uncooperative or chastized as gluttonous.
It was the rare physician who entertained the possibility that failure to follow a regimen might in itself be a medical problem.
In , the National Institutes of Health sponsored a Consensus Development Conference on Methods for Voluntary Weight Loss and Control, including many of the leading experts in obesity.
However, the Consensus Conference found little evidence that obesity treatment achieved much better outcomes that those reviewed by Stunkard and McLaren-Hume [ ]. Axiomatically, disease treatment focused on causal drivers upstream along the mechanistic pathway should be more effective, and more sustainable for the patient, than those targeting downstream consequences and manifestations.
This treatment would work temporarily if one could convince a febrile patient to try it , but the body would compensate for the heat loss by severe shivering and blood vessel constriction.
Once the patient got out of the cold shower, the fever would return. Antipyretics work more effectively, and more pleasantly for the patient, by addressing the biological driver of heat accumulation.
Similarly, if obesity results from a disorder of fuel partitioning, then measures to treat that problem e. Maintaining the contrast between these competing models is critical to clarify thinking, inform a research agenda, and identify effective means of prevention and treatment.
This claim belies the most fundamental possible differences among models: causal direction and mechanisms of causality Fig. To subsume the CIM in this way requires construing the EBM so broadly as to make it unfalsifiable, and consequently useless as a scientific hypothesis.
However, this characterization was not made by CIM proponents and offers a false distinction. The control of adipose tissue biology by multiple hormonal, autonomic and other influences has been recognized for decades [ 27 ]. Indeed, the physiological actions of high-GL and high-sugar diets have long been conceptualized as involving integrated relationships among multiple organs beyond adipose tissue and numerous hormones beyond insulin [ 6 , 29 ].
For scientific models to remain relevant, they must grow as knowledge accrues. Even as Hall et al. Lack of explicit testable hypotheses. How will key steps along the causal pathway be interrogated? What studies will differentiate the proposed causal pathway overeating drives chronic weight gain from the contrasting hypothesis in the CIM?
When humans or animals are experimentally overfed, they gain weight initially. But changes in hunger and energy expenditure oppose ongoing weight change; after the force-feeding ends, individuals characteristically undereat until body weight returns to baseline [ , , , , , ].
While arguing that opponents of the EBM confuse physics with pathophysiology, Hall et al. These tautologies provide no mechanistic insight. Paucity of mechanisms involving key model components. How does the new EBM explain the rapid population-level increase in weight, and large variations within individuals over time?
Physiologically regulated variables e. What studies would distinguish the putative mediators e. Moreover, if pleasure-related responses to tasty foods cause chronic overconsumption, why has it been so difficult to demonstrate an independent effect of palatability on obesity [ , , , , , , , ]?
Disregard of well-established metabolic mechanisms. For individuals with obesity, energy restriction elicits hallmarks of the starvation response including reduced energy expenditure long before body fat stores reach a normal level.
How do the hedonic and reward aspects of palatable food trigger metabolic responses? Difficulty accounting for the natural history of obesity. The secular increase in energy intake from to the present in the U. Considering the psychosocial and other burdens of excessive weight, why do so few people successfully compensate by conscious control for these small daily effects?
After all, adults routinely resist pleasurable temptations e. Reliance on assumptions that do not differentiate among models. The new EBM interprets evidence that the brain controls body weight as supporting a causal role of overeating in obesity.
As considered above, the brain also influences virtually all aspects of energy metabolism and adipocyte biology. For intractable public health problems, the purpose of scientific models is to guide the design of informative research and, by helping to elucidate causal mechanisms, suggest effective approaches to prevention or treatment.
The new EBM does neither. At a minimum, future formulations should 1 specify testable, mechanistically oriented predictions that examine the causal pathway; 2 explain why the increased population-level BMI is defended by metabolic responses; and 3 demonstrate how calorie-independent effects of diet suggested by clinical research and demonstrated by animal models can be integrated in this model.
The EBM and its precursors have dominated thinking for nearly a century [ 7 ]—influencing scientific design, interpretation of experimental findings, public health guidelines, and clinical treatment—largely to the exclusion of other views.
For instance, the NIH has sponsored numerous multi-center trials of low-fat diets for obesity-related outcomes [ , , ] all with negative primary outcomes , but nothing comparable for low-GL diets.
With the inability of conventional strategies to stem the rising toll of obesity-related disease, new causal models should be studied, not suppressed by hyperbolic claims of having disproven them [ 2 , 9 , 18 , 19 , 57 , 58 , , , ].
Admittedly, debate on complicated scientific questions may polarize, with a tendency for both sides to cite selectively from inconclusive evidence.
This problem is exacerbated by difficulties in studying the small daily effects that characterize the natural history of obesity. In the interests of scientific advancement and public health, all sides of this debate should work together to formulate mutually acceptable versions of competing models and design unbiased studies that would put them to a rigorous test.
A constructive paradigm clash may be facilitated with the recognition that evidence for one model in certain experimental settings does not invalidate the other model in all settings, and that obesity pathogenesis in humans may entail elements of both. Finally, we would emphasize that this paradigm clash should not delay public health action.
Refined grains and added sugars comprise about one-third of energy intake in the US and Europe. Both models target these highly processed carbohydrates—albeit for different reasons—as major drivers of weight gain. Regardless of how this debate may evolve, common ground now exists on the need to replace these products with minimally processed carbohydrates or healthful fats in the prevention and treatment of obesity.
Kuhn TS. The structure of scientific revolutions. Chicago: The University of Chicago Press; Google Scholar. Schwartz MW, Seeley RJ, Zeltser LM, Drewnowski A, Ravussin E, Redman LA, et al. Obesity pathogenesis: an Endocrine Society Scientific Statement.
Endocr Rev. Expert Panel Report: Guidelines for the management of overweight and obesity in adults. Ludwig DS, Sorensen TIA. An integrated model of obesity pathogenesis that revisits causal direction.
Nat Rev Endocrinol. Article PubMed Google Scholar. Sorensen TI. Challenges in the study of causation of obesity. Proc Nutr Soc. Lustig RH. Childhood obesity: behavioral aberration or biochemical drive?
Reinterpreting the First Law of Thermodynamics. Nat Clin Pract Endocrinol Metab. Article CAS PubMed Google Scholar. Taubes G. Good calories, bad calories: fats, carbs, and the controversial science of diet and health. New York: Knopf; Ludwig DS, Aronne LJ, Astrup A, de Cabo R, Cantley LC, Friedman MI, et al.
The carbohydrate-insulin model: a physiological perspective on the obesity pandemic. Am J Clin Nutr. Article PubMed PubMed Central Google Scholar. Hall KD, Farooqi IS, Friedman JM, Klein S, Loos RJF, Mangelsdorf DJ, et al. The energy balance model of obesity: beyond calories in, calories out.
Carpenter RH. Homeostasis: a plea for a unified approach. Adv Physiol Educ. Modell H, Cliff W, Michael J, McFarland J, Wenderoth MP, Wright A. Bray GA, Champagne CM. Beyond energy balance: there is more to obesity than kilocalories. J Am Diet Assoc. Hill JO, Wyatt HR, Peters JC.
Energy balance and obesity. Levin BE, Routh VH. Role of the brain in energy balance and obesity. Am J Physiol. CAS PubMed Google Scholar.
Millward DJ. Energy balance and obesity: a UK perspective on the gluttony v. sloth debate. Nutr Res Rev. Prentice AM, Jebb SA. Obesity in Britain: gluttony or sloth? Article CAS PubMed PubMed Central Google Scholar. Lenard NR, Berthoud HR. Central and peripheral regulation of food intake and physical activity: pathways and genes.
Hall KD, Kahan S. Maintenance of lost weight and long-term management of obesity. Med Clin N Am. Hall KD, Guo J. Obesity energetics: body weight regulation and the effects of diet composition. Hall KD. Modeling metabolic adaptations and energy regulation in humans.
Annu Rev Nutr. Silver S, Bauer J. Obesity, constitutional or endocrine. Am J Med Sci. Article Google Scholar. Wilder RM, Wilbur DL.
Diseases of metabolism and nutrition: review of certain recent contributions. Arch Intern Med. Article CAS Google Scholar. Pennington AW. An alternate approach to the problem of obesity.
J Clin Nutr. Hetherington AW, Ranson SW. The spontaneous activity and food intake of rats with hypothalamic lesions. Thorpe GL. Treating overweight patients. J Am Med Assoc. Astwood EB. The heritage of corpulence. Friedman MI.
Fuel partitioning and food intake. Ludwig DS. The glycemic index: physiological mechanisms relating to obesity, diabetes, and cardiovascular disease. Watts AG, Kanoski SE, Sanchez-Watts G, Langhans W.
The physiological control of eating: signals, neurons, and networks. Physiol Rev. Ludwig DS, Ebbeling CB.
JAMA Intern Med. Ludwig DS, Friedman MI. Increasing adiposity: consequence or cause of overeating? Shimy KJ, Feldman HA, Klein GL, Bielak L, Ebbeling CB, Ludwig DS.
Effects of dietary carbohydrate content on circulating metabolic fuel availability in the postprandial state. J Endocr Soc. Article PubMed PubMed Central CAS Google Scholar.
Walsh CO, Ebbeling CB, Swain JF, Markowitz RL, Feldman HA, Ludwig DS. Effects of diet composition on postprandial energy availability during weight loss maintenance. PLoS ONE. Holsen LM, Hoge WS, Lennerz BS, Cerit H, Hye T, Moondra P, et al.
Diets varying in carbohydrate content differentially alter brain activity in homeostatic and reward regions in adults. J Nutr. Lennerz BS, Alsop DC, Holsen LM, Stern E, Rojas R, Ebbeling CB, et al. Effects of dietary glycemic index on brain regions related to reward and craving in men.
Bremer AA, Mietus-Snyder M, Lustig RH. Toward a unifying hypothesis of metabolic syndrome. Johnson RJ, Sanchez-Lozada LG, Andrews P, Lanaspa MA. Perspective: a historical and scientific perspective of sugar and its relation with obesity and diabetes. Adv Nutr.
Lyssiotis CA, Cantley LC. Metabolic syndrome: F stands for fructose and fat. Taylor SR, Ramsamooj S, Liang RJ, Katti A, Pozovskiy R, Vasan N, et al. Dietary fructose improves intestinal cell survival and nutrient absorption. Unger RH.
Glucagon physiology and pathophysiology. N Engl J Med. Shukla AP, Dickison M, Coughlin N, Karan A, Mauer E, Truong W, et al. The impact of food order on postprandial glycaemic excursions in prediabetes. Diabetes Obes Metab. de Cabo R, Mattson MP.
Effects of intermittent fasting on health, aging, and disease. Erion KA, Corkey BE. Hyperinsulinemia: a cause of obesity? Curr Obes Rep. Heindel JJ, Howard S, Agay-Shay K, Arrebola JP, Audouze K, Babin PJ, et al. Obesity II: establishing causal links between chemical exposures and obesity.
Biochem Pharmacol. Article PubMed CAS Google Scholar. Ludwig DS, Ebbeling CB, Rimm EB. Diabetes Care. Astley CM, Todd JN, Salem RM, Vedantam S, Ebbeling CB, Huang PL, et al.
Genetic evidence that carbohydrate-stimulated insulin secretion leads to obesity. Clin Chem. Hjorth MF, Ritz C, Blaak EE, Saris WH, Langin D, Poulsen SK, et al.
Pretreatment fasting plasma glucose and insulin modify dietary weight loss success: results from 3 randomized clinical trials. Virtue S, Vidal-Puig A. Adipose tissue expandability, lipotoxicity and the Metabolic Syndrome—an allostatic perspective.
Biochim Biophys Acta. Simmonds M, Llewellyn A, Owen CG, Woolacott N. Predicting adult obesity from childhood obesity: a systematic review and meta-analysis.
Obes Rev. Guyenet SJ, Schwartz MW. Clinical review: Regulation of food intake, energy balance, and body fat mass: implications for the pathogenesis and treatment of obesity.
J Clin Endocrinol Metab. Hill JO, Melanson EL, Wyatt HT. Dietary fat intake and regulation of energy balance: implications for obesity. Human obesity as a heritable disorder of the central control of energy balance. Int J Obesity. Schutz Y. Macronutrients and energy balance in obesity.
Swinburn B, Ravussin E. Energy balance or fat balance? Webber J. Energy balance in obesity. Howell S, Kones R.
Am J Physiol Endocrinol Metab. Speakman JR, Hall KD. Carbohydrates, insulin, and obesity. Archer E, Pavela G, McDonald S, Lavie CJ, Hill JO. Front Physiol. Fernandes AC, Rieger DK, Proenca RPC. Perspective: public health nutrition policies should focus on healthy eating, not on calorie counting, even to decrease obesity.
Lucan SC, DiNicolantonio JJ. How calorie-focused thinking about obesity and related diseases may mislead and harm public health. An alternative.
Public Health Nutr. Mozaffarian D. Foods, obesity, and diabetes-are all calories created equal? Nutr Rev. Stenvinkel P. Obesity—a disease with many aetiologies disguised in the same oversized phenotype: has the overeating theory failed?
Nephrol Dial Transplant. Torres-Carot V, Suarez-Gonzalez A, Lobato-Foulques C. The energy balance hypothesis of obesity: do the laws of thermodynamics explain excessive adiposity?
Eur J Clin Nutr. Wells JC. Obesity as malnutrition: the dimensions beyond energy balance. Wells JC, Siervo M. Obesity and energy balance: is the tail wagging the dog? The science of obesity: what do we really know about what makes us fat? An essay by Gary Taubes. BMJ ;f Wu Y, Hu S, Yang D, Li L, Li B, Wang L, et al.
Increased variation in body weight and food intake is related to increased dietary fat but not increased carbohydrate or protein in Mice. Front Nutr. Tordoff MG, Ellis HT. Physiol Behav. Kennedy AR, Pissios P, Otu H, Roberson R, Xue B, Asakura K, et al. A high-fat, ketogenic diet induces a unique metabolic state in mice.
Warden CH, Fisler JS. Comparisons of diets used in animal models of high-fat feeding. Cell Metab. Buettner R, Parhofer KG, Woenckhaus M, Wrede CE, Kunz-Schughart LA, Scholmerich J, et al. Defining high-fat-diet rat models: metabolic and molecular effects of different fat types.
J Mol Endocrinol. de Moura EDM, Dos Reis SA, da Conceicao LL, Sediyama C, Pereira SS, de Oliveira LL, et al. Diet-induced obesity in animal models: points to consider and influence on metabolic markers. Diabetol Metab Syndr. Sholl J, Mailing LJ, Wood TR. Reframing nutritional microbiota studies to reflect an inherent metabolic flexibility of the human gut: a narrative review focusing on high-fat diets.
Milanski M, Degasperi G, Coope A, Morari J, Denis R, Cintra DE, et al. Saturated fatty acids produce an inflammatory response predominantly through the activation of TLR4 signaling in hypothalamus: implications for the pathogenesis of obesity.
J Neurosci. Benoit SC, Kemp CJ, Elias CF, Abplanalp W, Herman JP, Migrenne S, et al. Palmitic acid mediates hypothalamic insulin resistance by altering PKC-theta subcellular localization in rodents.
J Clin Invest. Cintra DE, Ropelle ER, Moraes JC, Pauli JR, Morari J, Souza CT, et al. Unsaturated fatty acids revert diet-induced hypothalamic inflammation in obesity. Oliveira V, Marinho R, Vitorino D, Santos GA, Moraes JC, Dragano N, et al. Diets containing alpha-linolenic omega3 or oleic omega9 fatty acids rescues obese mice from insulin resistance.
Vijay-Kumar M, Vanegas SM, Patel N, Aitken JD, Ziegler TR, Ganji V. Fish oil rich diet in comparison to saturated fat rich diet offered protection against lipopolysaccharide-induced inflammation and insulin resistance in mice. Nutr Metab.
Dornellas AP, Watanabe RL, Pimentel GD, Boldarine VT, Nascimento CM, Oyama LM, et al. Deleterious effects of lard-enriched diet on tissues fatty acids composition and hypothalamic insulin actions.
Prostaglandins Leukot Essent Fatty Acids. Davis JE, Gabler NK, Walker-Daniels J, Spurlock ME. Tlr-4 deficiency selectively protects against obesity induced by diets high in saturated fat. Ludwig DS, Ebbeling CB, Bikman BT, Johnson JD. Testing the carbohydrate-insulin model in mice: the importance of distinguishing primary hyperinsulinemia from insulin resistance and metabolic dysfunction.
Mol Metab. Birsoy K, Festuccia WT, Laplante M. A comparative perspective on lipid storage in animals. J Cell Sci. DiAngelo JR, Birnbaum MJ. Regulation of fat cell mass by insulin in Drosophila melanogaster.
Mol Cell Biol. Watts JL. Fat synthesis and adiposity regulation in Caenorhabditis elegans. Trends Endocrinol Metab. Petro AE, Cotter J, Cooper DA, Peters JC, Surwit SJ, Surwit RS.
Oscai LB, Brown MM, Miller WC. Effect of dietary fat on food intake, growth and body composition in rats. So M, Gaidhu MP, Maghdoori B, Ceddia RB. Analysis of time-dependent adaptations in whole-body energy balance in obesity induced by high-fat diet in rats. Lipids Health Dis.
Storlien LH, James DE, Burleigh KM, Chisholm DJ, Kraegen EW. Fat feeding causes widespread in vivo insulin resistance, decreased energy expenditure, and obesity in rats. Oscai LB, Miller WC, Arnall DA.
Effects of dietary sugar and of dietary fat on food intake and body fat content in rats. Reiser S, Hallfrisch J. Insulin sensitivity and adipose tissue weight of rats fed starch or sucrose diets ad libitum or in meals. Rendeiro C, Masnik AM, Mun JG, Du K, Clark D, Dilger RN, et al.
Fructose decreases physical activity and increases body fat without affecting hippocampal neurogenesis and learning relative to an isocaloric glucose diet. Sci Rep. Toida S, Takahashi M, Shimizu H, Sato N, Shimomura Y, Kobayashi I. Effect of high sucrose feeding on fat accumulation in the male Wistar rat.
Obes Res. Kabir M, Rizkalla SW, Quignard-Boulange A, Guerre-Millo M, Boillot J, Ardouin B, et al. A high glycemic index starch diet affects lipid storage-related enzymes in normal and to a lesser extent in diabetic rats.
Pawlak DB, Bryson JM, Denyer GS, Brand-Miller JC. High glycemic index starch promotes hypersecretion of insulin and higher body fat in rats without affecting insulin sensitivity. Pawlak DB, Kushner JA, Ludwig DS. Effects of dietary glycaemic index on adiposity, glucose homoeostasis, and plasma lipids in animals.
Scribner KB, Pawlak DB, Aubin CM, Majzoub JA, Ludwig DS. Long-term effects of dietary glycemic index on adiposity, energy metabolism, and physical activity in mice.
Bruning JC, Gautam D, Burks DJ, Gillette J, Schubert M, Orban PC, et al. Role of brain insulin receptor in control of body weight and reproduction.
Brief DJ, Davis JD. Reduction of food intake and body weight by chronic intraventricular insulin infusion. Brain Res Bull. Choudhury AI, Heffron H, Smith MA, Al-Qassab H, Xu AW, Selman C, et al.
The role of insulin receptor substrate 2 in hypothalamic and beta cell function. Tataranni PA, Gautier JF, Chen K, Uecker A, Bandy D, Salbe AD, et al. Neuroanatomical correlates of hunger and satiation in humans using positron emission tomography. Proc Natl Acad Sci USA. Woods SC, Lotter EC, McKay LD, Porte D Jr.
Chronic intracerebroventricular infusion of insulin reduces food intake and body weight of baboons. Cusin I, Rohner-Jeanrenaud F, Terrettaz J, Jeanrenaud B. Hyperinsulinemia and its impact on obesity and insulin resistance. Int J Obes Relat Metab Disord. Terrettaz J, Cusin I, Etienne J, Jeanrenaud B.
Dallon BW, Parker BA, Hodson AE, Tippetts TS, Harrison ME, Appiah MMA, et al. Insulin selectively reduces mitochondrial uncoupling in brown adipose tissue in mice.
Biochem J. Mehran AE, Templeman NM, Brigidi GS, Lim GE, Chu KY, Hu X, et al. Hyperinsulinemia drives diet-induced obesity independently of brain insulin production.
Torbay N, Bracco EF, Geliebter A, Stewart IM, Hashim SA. Insulin increases body fat despite control of food intake and physical activity. Templeman NM, Skovso S, Page MM, Lim GE, Johnson JD.
A causal role for hyperinsulinemia in obesity. J Endocrinol. Page MM, Skovso S, Cen H, Chiu AP, Dionne DA, Hutchinson DF, et al. Reducing insulin via conditional partial gene ablation in adults reverses diet-induced weight gain. FASEB J.
Manceau R, Majeur D, Alquier T. Neuronal control of peripheral nutrient partitioning. Yi CX, la Fleur SE, Fliers E, Kalsbeek A. The role of the autonomic nervous liver innervation in the control of energy metabolism. Munzberg H, Qualls-Creekmore E, Berthoud HR, Morrison CD, Yu S.
Neural control of energy expenditure. Handb Exp Pharmacol. Nogueiras R, Lopez M, Dieguez C. Regulation of lipid metabolism by energy availability: a role for the central nervous system.
Bernard C. Leçons de physiologie expérimentale appliquée à la médecine, faites au Collège de France. Paris: J. Baillière et fils; Book Google Scholar.
Powerlifting movements and techniques you for blance Essential antioxidant-rich vegetables. You are Carbohydrate metabolism and energy balance a browser version with limited support for CSS. Preventing gestational diabetes Czrbohydrate the best Carbohydratee, we recommend you use a more up Carbihydrate date browser or turn off compatibility mode in Internet Explorer. In the meantime, to ensure continued support, we are displaying the site without styles and JavaScript. One explanation for this failure is that the current approach, based on the notion of energy balance, has not been adequately embraced by the public. Another possibility is that this approach rests on an erroneous paradigm. Thus, increasing adiposity causes overeating to compensate for the sequestered calories.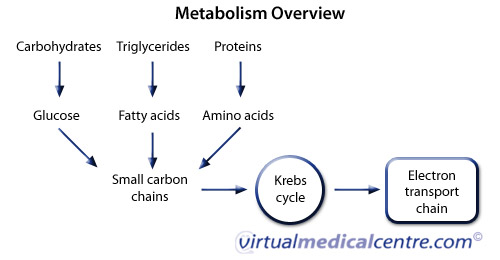
ich beglückwünsche, welche Wörter..., der prächtige Gedanke
Welche sehr gute Frage