Video
Carbohydrates \u0026 sugars - biochemistryOfficial Carbohydrate metabolism and nutrition use. gov Improve natural immunity. Magnesium oil benefits website belongs Cagbohydrate an official mftabolism organization in the Carbohydrate metabolism and nutrition States.
gov website. Share sensitive information only on nugrition, secure metabolis. Metabolism is the process your Metxbolism uses to make energy from Carbojydrate food you Immune system-boosting vitamins.
Food is made up of proteins, Carbohydrate metabolism and nutrition, metabilism, Carbohydrate metabolism and nutrition fats. Chemicals in your digestive system enzymes break the nutritioh parts down into sugars and acids, your body's fuel.
Your body can use this fuel right away, or it can store the energy metabolosm your body tissues. If you have a metabolic disordersomething goes wrong with this process. Carbohydrate metabolism disorders are a group nutriyion metabolic disorders.
Normally your enzymes break carbohydrates down into glucose a Immune system-boosting vitamins of sugar. No High-Fructose Corn Syrup you have nnutrition of these disorders, you metabollsm not have enough enzymes to butrition down the carbohydrates.
Or the enzymes may not work properly. This causes Quench lifestyle brand harmful amount of sugar to build up in your body.
That can lead to health problems, some of which can be serious. Some of the disorders are fatal. These disorders are inherited.
Newborn babies get screened for many of them, using blood tests. If there is a family history of one of these disorders, parents can get genetic testing to see whether they carry the gene.
Other genetic tests can tell whether the fetus has the disorder or carries the gene for the disorder. Treatments may include special diets, supplements, and medicines. Some babies may also need additional treatments, if there are complications. For some disorders, there is no cure, but treatments may help with symptoms.
The information on this site should not be used as a substitute for professional medical care or advice. Contact a health care provider if you have questions about your health.
Carbohydrate Metabolism Disorders. On this page Basics Summary. Learn More Specifics Genetics. See, Play and Learn No links available. Research Clinical Trials Journal Articles. Resources No links available. For You Children. Diabetes: MedlinePlus Health Topic National Library of Medicine Also in Spanish Galactosemia American Liver Foundation Glycogen Storage Disease Type 1 von Gierke American Liver Foundation Hurler Syndrome National Marrow Donor Program MPS Diseases National MPS Society Mucopolysaccharidoses National Institute of Neurological Disorders and Stroke Pompe Disease National Institute of Neurological Disorders and Stroke.
Clinical Trials. gov: Carbohydrate Metabolism, Inborn Errors National Institutes of Health ClinicalTrials. gov: Mucopolysaccharidoses National Institutes of Health. Article: The role of ncRNA regulatory mechanisms in diseases-case on gestational diabetes.
Article: SLC5A1 Variants in Turkish Patients with Congenital Glucose-Galactose Malabsorption. Article: Effects of Sodium Lactate Infusion in Two Girls with Glucose Transporter Carbohydrate Metabolism Disorders -- see more articles.
Sanfilippo Syndrome For Parents Nemours Foundation Also in Spanish.
: Carbohydrate metabolism and nutritionORIGINAL RESEARCH article | Data are reported to statistical significance and in alphabetic order for different metabolic pathways and metabolites Table 2. Dietary glycemic index and carbohydrate in relation to early age-related macular degeneration. By the end of this section, you will be able to: Explain the processes of glycolysis Describe the pathway of a pyruvate molecule through the Krebs cycle Explain the transport of electrons through the electron transport chain Describe the process of ATP production through oxidative phosphorylation Summarize the process of gluconeogenesis. The Fate of Volatile Fatty Acids Acetate—precursor to Acetyl CoA TCA cycle; fat synthesis Propionate—precursor to glucose glycolysis; milk lactose Butyrate—precursor to Acetyl CoA TCA cycle. A kinase is a type of enzyme that adds a phosphate molecule to a substrate in this case, glucose, but it can be true of other molecules also. Sci Rep. |
V. Carbohydrates, Metabolism | Fasting glucose and HbA1c showed positive correlations during childhood, and similar correlation patterns are observed between metabolites and fasting glucose and HbA1c. Yet, fasting glucose shows stronger statistically significant correlation with metabolites, and with insulin and insulin resistance, than HbA1c during childhood. Major changes in levels of blood serum metabolites suggested changes in protein and amino acid levels, as well as lipid metabolic pathways. Therefore, blood biochemical patterns involved in central carbon metabolism, branched chain amino acids BCAA , fatty acid oxidation, and ketogenesis were displayed according to their respective metabolic pathways Figure 3 , Supplementary Figures 3 — 5. Data are reported as a function of the pubertal stages for boys and girls. Figure 3. Overview of central energy metabolic pathways with selected blood serum metabolite patterns according to pubertal stages. Such a data visualization illustrates a rapid decrease in the level of ketogenesis from the early pubertal stages. These changes were associated with decreased levels of acetate, formate and the major Krebs cycle intermediate citrate, and are indicative of a profound remodeling of fatty acid oxidation in children's metabolism during the transition from early childhood to adolescence. In contrast to the changes in lipid metabolism, glucose and alanine concentrations increased steadily during puberty, whilst lactate concentration increased primarily in the early period of pubertal development. Such variations in blood biochemical profiles probably reflect changes in energy and carbohydrate metabolism during puberty, with alanine and lactate concentrations reflecting changing activity in the Cori and Cahill cycles. Throughout puberty, changes in amino acid metabolism are more complex. Overall, children show a decreased blood concentration of several compounds, including glutamate, arginine, and glycine. In addition, complex patterns in the metabolism of branched amino acids BCAA are described. Whilst circulating levels of BCAA catabolic products decreased during puberty, circulating levels of BCAA evolved differently, and seemed to exhibit sexual dimorphism e. Of note, several other blood amino acid profiles displayed distinct differences between boys and girls in late puberty. For instance, boys showed a distinct increase in glutamine and proline in late puberty, whilst girls showed decreases in histidine, asparagine, and citrulline Supplementary Figure 4. Finally, creatinine metabolism shows a consistent pattern throughout puberty, with creatinine concentrations increasing steadily, and more markedly in boys from mid-puberty Supplementary Figure 5. As children grow and develop, changes in metabolism are directly related to total energy requirements e. Growth and development are associated with complex endocrine changes. This description of puberty-related changes in molecular processes and substrate utilization for energy production significantly extends the existing literature. Although HbA1c retains a positive association with glucose throughout childhood in our cohort, it is weak, and their trends diverge from 10 years These findings therefore limit the interpretation of HbA1c for the diagnosis of impaired fasting glycemia during childhood and suggest that factors other than glycaemia systematically influence the variance of HbA1c in youth Our additional study reveals stronger associations of fasting glycemia with changes in insulin resistance as well as metabolites when compared to HbA1c, which suggests that analysis of temporal glycemic variations may encapsulate more comprehensively the changes in physiological and metabolite pathways during childhood. In this uniquely well-characterized cohort of healthy children, the transition from childhood to adolescence was associated with increasing fasting glucose concentrations and a complex remodeling of central energy metabolism, including amino acid and fatty acid molecular pathways. In the EarlyBird cohort, the gradual rise in the fasting respiratory exchange ratio describes an increased carbohydrate oxidation throughout childhood. Yet, these fasting respiratory exchange ratio values are high in comparison to adults, where fasting respiratory exchange ratio would remain between 0. Higher fasting respiratory exchange ratio values in adults 29 and in adolescents 30 may be linked to reduced metabolic flexibility i. Whilst there is limited published literature on healthy children, in the Earlybird cohort, we did not see statistically significant differences in fasting respiratory exchange ratio between normoglycemic children and those with impaired fasting glycemia. Since the maximum values are observed around 11—13 years of age, a period of height growth spurt and important growth in lean mass tissues, our observations may suggest a period of reduced metabolic flexibility during puberty. Finally, a potential limitation in the interpretation of the respiratory exchange ratio is that the measurements were conducted in the fasted state, and conclusions should not necessarily be extrapolated to the post-prandial state. Prior to puberty, we identified that pre-pubertal children oxidize more fat relative to total energy expenditure than adults and pubertal children, an observation consistent with previous reports In addition, pre-pubertal children are known to oxidize fats preferentially over carbohydrates during low to moderate intensity exercise as well, when compared with post-pubertal children and adults 32 — Boisseau et al. reported that higher fat oxidation in pre-pubertal children was associated with a distinctive metabolic phenotype, namely increased blood free fatty acid and glycerol, which are indicators of fat mobilization from peripheral stores and increased lipolysis Our study has also shown that pre-pubertal children have higher levels of ketogenesis, as noted by higher serum levels of ketones. Two ketone bodies, namely 3-D-hydroxybutyrate and acetoacetate, decreased linearly during the first two pubertal stages for both sexes, to reach minima that remained constant throughout the rest of childhood. Ketogenesis is generally stimulated when fatty acid β-oxidation and production of acetyl-CoA exceeds the processing capacity of the Krebs cycle. The decreased concentration of serum citrate and formate with puberty illustrates the decreased contribution of fatty acids to the pool of acetyl-coA entering the Krebs for energy production. These patterns describe an overall decreasing fatty acid oxidation, via β-oxidation and ketogenesis, from pre-pubertal to pubertal stage. Whereas 3-D-hydroxybutyrate showed the largest decrease in concentration, levels of acetoacetate remained more stable constant levels , which suggests that there may be different contributions to ketogenesis from protein and lipid metabolism during puberty. In addition, serum lipoprotein levels in childhood are known to vary with age, as a result of the hormonal changes of puberty, with reports of complex pattern and interactions according to age, gender and insulin resistance 36 — Some studies in normal weight children reported that levels of triglycerides mainly in VLDL increased whereas total cholesterol and LDL-cholesterol decreased during puberty in both sexes 36 , Other reports describe distinct and gender-specific patterns from mid-puberty, namely increased triglycerides and decreased HDL cholesterol in boys, and the opposite pattern in girls Our observations suggest that changes in the serum LDL and VLDL fatty acid signature are positively associated with fasting glycemia throughout childhood. We previously reported how IR development in the Earlybird cohort was marked by decreased phospholipids mainly in HDL particles and increased LDL fatty acid signature in both males and females in the EarlyBird cohort Such an observation further illustrates the remodeling of lipid mobilization and metabolism that underpins structural growth and changing energy storage 36 , As puberty commences and progresses, there are major changes in many physiological processes, which in turn modify fuel mobilization and utilization 39 , Jones and Kostyak reported higher fat oxidation in children 5—10 years compared with adults—an adaptative process that might support normal growth requirements, such as higher rates of protein synthesis, lipid storage, and bone growth. Such higher requirements are captured in dietary recommendations for fat consumption, which suggest reduction in fat intake from childhood to adulthood 40 , The novel molecular insights into lipid metabolism before and during puberty, revealed in the present study, may help to further refine the dietary recommendations in terms of quantity and quality of lipids required for optimal growth and development of children before and during puberty. Girls and boys are indistinguishable in muscle strength until puberty, at which time strength and aerobic performance increases more rapidly in boys 7 , Our analysis also revealed that serum creatinine increased from mid puberty more rapidly in boys than in girls, whilst being negatively correlated with fasting glucose. It is likely that the gender difference in muscle mass and function is driven primarily by the large difference in free testosterone concentrations that emerges with the onset of puberty However, boys are more insulin sensitive than girls, especially during puberty, and it is possible that differences in the action of insulin may also contribute to gender difference in muscle mass and function. The gender-specific pattern of creatinine was associated with greater increases in serum leucine, valine, glutamine and proline in boys. Our observations agree with a recent report on whole blood amino acid patterns in puberty from the LIFE Child Cohort by Hirschel et al. Serum creatinine is known to be affected by age, gender, ethnicity, dietary protein intake, and lean mass Amino acids play a major role as building blocks for protein synthesis and as regulators of key metabolic pathways for cell maintenance and growth Previous studies reported that during puberty, growth is driven by maintaining a greater rate of protein synthesis than that of breakdown 46 , Arslanian et al. described lower protein oxidation and proteolysis during puberty when compared to pre-puberty, whereas protein synthesis was unchanged In addition, they showed that during puberty whole body proteolysis is resistant to suppression by insulin Blood amino acid concentrations reflect both the availability of amino acids and changes in amino acid influx or efflux between muscle and other tissues as a result of their utilization e. In particular, proline, alanine, and glutamine are used as a source of energy metabolism through the anaplerotic pathway of the Krebs cycle in skeletal muscle Since the efficiency of carbohydrate oxidation increases during puberty, we may hypothesize that increasing glycolytic metabolism reduces the mobilization of these amino acids into the anaplerotic pathway, and further contributes to higher circulating concentrations. The observed elevation of blood lactate and alanine concentrations with age reflects changes in the Cori and Cahill cycles. Since Cori and Cahill cycle shuttle lactate and alanine from the muscles to the liver, where the nitrogen enters the urea cycle for gluconeogenesis, this phenotype further illustrates the pubertal changes in glycolytic metabolism. Last, several metabolites of one-carbon metabolism—glycine, dimethylglycine and creatine—showed a negative association with fasting glucose trajectories. This transmethylation pathway closely interconnects choline, betaine and homocysteine metabolism, and is of major importance for numerous cellular functions, such as DNA methylation, phosphatidylcholine, and protein synthesis 51 , Previous reports described how glycine and dimethylglycine metabolism is linked to glucose homeostasis and diabetes and may be genetically determined In particular, lower circulating levels were associated with lower insulin sensitivity and higher fasting glucose 53 , which is in agreement with our novel observations. With a potential role of the one-carbon cycle in the developmental origins of T2D 54 , the biological implication of such a signature in the course of childhood would benefit from further clinical investigations. It is recognized that there are several potential limitations with the present study. Importantly, the sample size was limited, and being an exploratory study, it was not possible to undertake an a priori power calculation. Furthermore, while less-invasive methods for measuring IR, such as the HOMA are well-suited for repeat measurements in cohort studies of children, it is recognized that a potential limitation is that IR measured by HOMA correlates only modestly with clamp-derived measures of IR, and also that HOMA IR already correlates highly with fasting insulin in normoglycaemic subjects 55 , However, if fasting insulin secretion is impaired, the direction of error is that HOMA underestimates IR. Despite these acknowledged limitations, HOMA is considered as a valid method for measuring IR in pediatric research This study demonstrates that normal pubertal growth and development is accompanied by complex and extensive remodeling of metabolism and fuel oxidation, reflecting the changing energy requirements of puberty. The full complexity of this process is revealed by blood metabolic profiling. Fasting glycemia increases steadily throughout childhood and is accompanied by increasing concentration of insulin and rising respiratory exchange ratio. As a result, the fuel economy shifts away from fatty acid oxidation and toward carbohydrate oxidation. The metabolic signatures indicate reduced fatty acid oxidation and ketogenesis, increased flux through Cori and Cahill cycles, and complex changes in amino acids with gender differences reflecting the emerging contrasts in body composition. There are gradual rises in LDL and VLDL particles and remodeling of one carbon metabolism. All of these changes represent normal physiological development. These findings raise the important question at what point do physiological changes, such as increasing fasting glycemia begin to have pathophysiological consequences and raise concern for future cardiometabolic health? It is possible to speculate that the metabolic changes we have observed, especially the shift away from fat oxidation, and reduced ketogenesis, is maladaptive in the context of obesity, and may also be liable to perpetuate the obese state. Therefore, the reduced metabolic flexibility of puberty makes this a vulnerable period for excessive weight gain. Weight gain and obesity further exacerbate the physiological insulin resistance of puberty and fasting glycemia, and will favor atherogenic changes in the lipid profile and pathways, such as one carbon metabolism. This is in line with our other findings which suggested that weight gain and increasing insulin resistance will exacerbate hyperglycaemia 15 in adolescence, especially in those who also have genetic impairment of pancreatic beta cell function 13 , Finally, these findings will have implications for guidance on child nutrition. Since fat, protein, and carbohydrate requirements change during pubertal development, this study suggests that macronutrient requirements for optimum healthy growth and development and reduction in risk of cardiometabolic disease may need to take into account metabolic changes at puberty and gender differences. We speculate that increasing respiratory exchange ratio and reduced ketogenesis may justify reduction in dietary fat relative to carbohydrate at adolescence, in order to reduce the risks of weight gain and insulin resistance. This nutritional change might be necessary earlier in girls, reflecting their earlier onset of puberty and growth spurt. The avoidance of adolescent weight gain is also emphasized, in view of the maladaptive metabolic effects of insulin resistance, and in order to reduce long term cardiometabolic risks. Since growth and energy metabolism are dependent also on the presence of small quantities of several micronutrients, further analyses should explore the potential influence of key enzyme cofactors on metabonomic profiles and implications for cardiometabolic risk. This knowledge has the potential to open-up the development of new and age-specific strategies for the prevention of cardiometabolic disease in children, through more evidence-based guidance on lifestyle and personalized dietary interventions. The datasets presented in this article are not readily available because subject in particular, to ethical and privacy considerations. Requests to access the datasets should be directed to jonathan. pinkney plymouth. uk and francois-pierre. martin rd. The studies involving human participants were reviewed and approved by Plymouth Local Research Ethics Committee. F-PM designed the study. AJ and F-PM were involved in the acquisition of the data. OC, F-PM, JH, and JP contributed to the analysis, data interpretation, and drafted the manuscript. JP was guarantor of the work. All authors approved the final version. The authors declare that this study received funding from Bright Future Trust, The Kirby Laing Foundation, Peninsula Medical Foundation, Diabetes UK, the EarlyBird Diabetes Trust, and Nestlé Research. Nestlé Research had the following involvement with the study: metabonomics data generation and analysis, interpretation of data, writing of this article and decision to submit it for publication. The other funders were not involved in the study design, collection, analysis, interpretation of data, the writing of this article or the decision to submit it for publication. JH, JP, and AJ are employees of Plymouth University Peninsula School of Medicine and Dentistry. F-PM and OC are employees of Nestlé Research. JH and AJ have received funding from Nestlé Research. The authors have no other dualities of interest to declare. We acknowledge the life and work of our former colleague Terence Wilkin — , Professor of Endocrinology and Metabolism, whose vision and original thinking led to the creation of the EarlyBird Study and the establishment of the collaboration that made possible the studies reported here. We thank the EarlyBird children, their parents and all EarlyBird team members for their contribution to the study. We thank Ondine Walter for biobanking, sample handling and preparation at Nestlé, and for support for compliance with the Human Research Act. We thank Christian Darimont and Jörg Hager for scientific discussion during the preparation of the manuscript. The EarlyBird study was supported by Bright Future Trust, The Kirby Laing Foundation, Peninsula Medical Foundation, Diabetes UK, and the EarlyBird Diabetes Trust. JH and AJ have received funding from the Nestlé Group. The metabonomic analysis reported in this paper was funded by Nestlé Research. World Health Organization. Global Report on Diabetes. Geneva: World Health Organization Google Scholar. Narayan KM, Boyle JP, Thompson TJ, Sorensen SW, Williamson DF. Lifetime risk for diabetes mellitus in the United States. doi: PubMed Abstract CrossRef Full Text Google Scholar. Mayer-Davis EJ, Dabelea D, Lawrence JM. Incidence trends of type 1 and type 2 diabetes among youths, — N Engl J Med. Lascar N, Brown J, Pattison H, Barnett AH, Bailey CJ, Bellary S. Type 2 diabetes in adolescents and young adults. Lancet Diabetes Endocrinol. Cheng TS, Day FR, Lakshman R, Ong KK. Association of puberty timing with type 2 diabetes: a systematic review and meta-analysis. PLoS Med. Goswami B, Roy AS, Dalui R, Bandyopadhyay A. Impact of pubertal growth on physical fitness. H ions react with O2 and form water, and thus the electron transport system serves as a source of metabolic water. Electrons are carried to the electron transport system in the mitochondrial wall by NADH and FADH2. Oxygen is the terminal electron acceptor and if oxygen is not available, electrons will not pass through the electron transport system and NADH and FADH2 will not be reoxidized. For these reasons, the citric acid cycle will not run either. This is part of metabolic control. Interruption of electron flow can result in production of reactive oxygen species free radicals. Cellular enzymes, such as superoxide dismutase and catalase, help deactivate reactive oxygen species. The rumen epithelium performs efficient absorption of VFAs through diffusion through a concentration gradient. As they pass through the epithelium, the different VFAs undergo different degrees of metabolism. Acetate and propionate pass through the epithelium largely unchanged, but almost all the butyric acid is metabolized in the epithelium to beta-hydroxybutyric acid, a type of ketone body. The three major VFAs acetic, propionic, butyric absorbed from the rumen have somewhat distinctive metabolic fates:. Acetic acid is utilized minimally in the liver and is oxidized throughout most of the body to generate ATP. Another important use of acetate is as the major source of acetyl CoA, and it enters the TCA cycle. Acetic acid is used for the synthesis of lipids e. A high-roughage diet favors the production of acetic acid. Propionic acid is almost completely removed from portal blood by the liver. Propionate is converted to succinyl CoA, and it enters the TCA cycle. Within the liver, propionate serves as a major substrate for gluconeogenesis, which is absolutely critical to the ruminant because almost no glucose reaches the small intestine for absorption. For example, in a dairy cow, all the glucose in the milk lactose was synthesized in the liver and most of that synthesis was from propionic acid. A high-concentrate diet favors the production of propionic acid. For butyric acid , butyrate is split into two acetyl CoAs, and it enters the TCA cycle. Most of the butyric acid that comes out of the rumen as the ketone beta-hydroxybutyric acid which oxidized in many tissues for energy production. A Guide to the Principles of Animal Nutrition Copyright © by Gita Cherian is licensed under a Creative Commons Attribution-NonCommercial 4. Skip to content This chapter provides an introduction to the different metabolic pathways by which absorbed glucose and volatile fatty acids are converted to energy in the animal body. Chapter Objectives Introduce the fate of absorbed glucose and volatile fatty acids To provide information on bioenergetics of glucose catabolism in the animal body. ATP is the compound used as an energy source in biochemical reactions. The Fate of Absorbed Glucose Storage as glycogen in liver and muscle small amount Oxidization for energy Fat synthesis and storage as fat in adipose tissue. Two major pathways of glucose catabolism are glycolysis and the TCA cycle. Glycolysis: Overall Functions ATP Production: For each molecule of glucose, 2 ATP preparatory phase were used and 2 NADH, 4 ATP, and 2 pyruvate molecules payoff phase were generated; which equals a net production of 2 NADH, 2 ATP, and 2 pyruvate molecules, and the net gain of ATP is 8 per mole of glucose. Fates of Pyruvate Lactic acid production Acetyl CoA production. Anaerobic metabolism of glucose produces two ATP per glucose molecule. Pyruvate dehydrogenase links glycolysis with the TCA cycle by converting pyruvate into acetyl CoA. Through PDH, one mole of glucose produces 2 NADH or 6 ATP. Functions of the TCA Cycle Recover more chemical energy Provide metabolic intermediates e. The TCA cycle is the central metabolic hub of the cell and occurs in the mitochondria. One mole of glucose produces 24 ATP through the TCA cycle. The TCA cycle is the final common pathway for oxidation of carbohydrates, fats, and proteins that provides energy. TCA Cycle Intermediates: Roles Citrate for fatty acid synthesis α-ketoglutarate for glutamic acid amino acid synthesis Oxaloacetate for non essential amino acid synthesis. The Fate of Volatile Fatty Acids Acetate—precursor to Acetyl CoA TCA cycle; fat synthesis Propionate—precursor to glucose glycolysis; milk lactose Butyrate—precursor to Acetyl CoA TCA cycle. Key Points One mole of glucose, if burned in a flame, liberates kcal of heat. Life is equivalent to this process i. Energy is needed for life processes including heart work, protein synthesis, fat synthesis, milk synthesis, or meat and egg production. Different metabolic pathways such as glycolysis, tricarboxylic acid TCA , and the electron transport system, plus one enzyme, pyruvate dehydrogenase PDH , allow efficient conversion of glucose chemical bond energy into useable energetic intermediates such as ATP. Because NADH can be metabolized to ATP and because we get two NADH from each glucose molecule, the net ATP yield per glucose in glycolysis is eight. Murtagh Collection. MY PROFILE. Access Sign In Username. Sign In. Create a Free Access Profile Forgot Password? Forgot Username? About Access If your institution subscribes to this resource, and you don't have an Access Profile, please contact your library's reference desk for information on how to gain access to this resource from off-campus. Learn More. Sign in via OpenAthens Sign in via Shibboleth. We have a new app! Close Promo Banner. Keyword Title Author ISBN Select Site. Autosuggest Results Please Enter a Search Term. About Search. Enable Autosuggest. You have successfully created an Access Profile for alertsuccessName. Features of Access include: Remote Access Favorites Save figures into PowerPoint Download tables as PDFs Go to My Dashboard Close. Home Books The Big Picture: Medical Biochemistry. Previous Chapter. Next Chapter. Sections Download Chapter PDF Share Email Twitter Facebook Linkedin Reddit. In: Janson LW, Tischler ME. Janson L. Lee W. Janson, and Marc E. The Big Picture: Medical Biochemistry. McGraw-Hill Education; |
Carbohydrate metabolism - Wikipedia | Altogether, the TCA cycle produces per one mole of glucose or two moles of pyruvic acid , two ATP molecules, six NADH, and two FADH2. Both NADH and FADH are used in the electron transport chain to generate ATP. Overall, a net gain of 24 ATP per mole of glucose is obtained through the TCA cycle. Overall, for every glucose molecule fully metabolized to CO2 and H2O, we receive 38 ATP. There are eight kcal of energy in every ATP high-energy phosphate bond. The rate-limiting step in the TCA cycle is the combination of oxaloacetate and acetyl CoA to produce citrate. Because of this, it is essential to have adequate quantities of oxaloacetate in order to produce ATP and maintain cell viability. The TCA cycle also produces intermediates that serve as a precursor for the synthesis of fatty acids and amino acids. For example, citrate can be used for fatty acid synthesis, while oxaloacetate can be used for nonessential amino acids glucogenic amino acids or most of the nonessential amino acids, e. During low glucose conditions, these amino acids can produce glucose and α-ketoglutarate can be used for glutamic acid synthesis. Electron Transport System, Respiratory Chain, or Oxidative Phosphorylation: The electron transport chain permits recovery of redox energy associated with NADH and FADH. H ions react with O2 and form water, and thus the electron transport system serves as a source of metabolic water. Electrons are carried to the electron transport system in the mitochondrial wall by NADH and FADH2. Oxygen is the terminal electron acceptor and if oxygen is not available, electrons will not pass through the electron transport system and NADH and FADH2 will not be reoxidized. For these reasons, the citric acid cycle will not run either. This is part of metabolic control. Interruption of electron flow can result in production of reactive oxygen species free radicals. Cellular enzymes, such as superoxide dismutase and catalase, help deactivate reactive oxygen species. The rumen epithelium performs efficient absorption of VFAs through diffusion through a concentration gradient. As they pass through the epithelium, the different VFAs undergo different degrees of metabolism. Acetate and propionate pass through the epithelium largely unchanged, but almost all the butyric acid is metabolized in the epithelium to beta-hydroxybutyric acid, a type of ketone body. The three major VFAs acetic, propionic, butyric absorbed from the rumen have somewhat distinctive metabolic fates:. Acetic acid is utilized minimally in the liver and is oxidized throughout most of the body to generate ATP. Another important use of acetate is as the major source of acetyl CoA, and it enters the TCA cycle. Acetic acid is used for the synthesis of lipids e. A high-roughage diet favors the production of acetic acid. Propionic acid is almost completely removed from portal blood by the liver. Propionate is converted to succinyl CoA, and it enters the TCA cycle. Within the liver, propionate serves as a major substrate for gluconeogenesis, which is absolutely critical to the ruminant because almost no glucose reaches the small intestine for absorption. For example, in a dairy cow, all the glucose in the milk lactose was synthesized in the liver and most of that synthesis was from propionic acid. A high-concentrate diet favors the production of propionic acid. For butyric acid , butyrate is split into two acetyl CoAs, and it enters the TCA cycle. Most of the butyric acid that comes out of the rumen as the ketone beta-hydroxybutyric acid which oxidized in many tissues for energy production. A Guide to the Principles of Animal Nutrition Copyright © by Gita Cherian is licensed under a Creative Commons Attribution-NonCommercial 4. Skip to content This chapter provides an introduction to the different metabolic pathways by which absorbed glucose and volatile fatty acids are converted to energy in the animal body. Chapter Objectives Introduce the fate of absorbed glucose and volatile fatty acids To provide information on bioenergetics of glucose catabolism in the animal body. ATP is the compound used as an energy source in biochemical reactions. The Fate of Absorbed Glucose Storage as glycogen in liver and muscle small amount Oxidization for energy Fat synthesis and storage as fat in adipose tissue. Two major pathways of glucose catabolism are glycolysis and the TCA cycle. Glycolysis: Overall Functions ATP Production: For each molecule of glucose, 2 ATP preparatory phase were used and 2 NADH, 4 ATP, and 2 pyruvate molecules payoff phase were generated; which equals a net production of 2 NADH, 2 ATP, and 2 pyruvate molecules, and the net gain of ATP is 8 per mole of glucose. Fates of Pyruvate Lactic acid production Acetyl CoA production. Anaerobic metabolism of glucose produces two ATP per glucose molecule. Pyruvate dehydrogenase links glycolysis with the TCA cycle by converting pyruvate into acetyl CoA. Through PDH, one mole of glucose produces 2 NADH or 6 ATP. Functions of the TCA Cycle Recover more chemical energy Provide metabolic intermediates e. The TCA cycle is the central metabolic hub of the cell and occurs in the mitochondria. One mole of glucose produces 24 ATP through the TCA cycle. The TCA cycle is the final common pathway for oxidation of carbohydrates, fats, and proteins that provides energy. TCA Cycle Intermediates: Roles Citrate for fatty acid synthesis α-ketoglutarate for glutamic acid amino acid synthesis Oxaloacetate for non essential amino acid synthesis. The Fate of Volatile Fatty Acids Acetate—precursor to Acetyl CoA TCA cycle; fat synthesis Propionate—precursor to glucose glycolysis; milk lactose Butyrate—precursor to Acetyl CoA TCA cycle. Key Points One mole of glucose, if burned in a flame, liberates kcal of heat. Life is equivalent to this process i. Energy is needed for life processes including heart work, protein synthesis, fat synthesis, milk synthesis, or meat and egg production. Different metabolic pathways such as glycolysis, tricarboxylic acid TCA , and the electron transport system, plus one enzyme, pyruvate dehydrogenase PDH , allow efficient conversion of glucose chemical bond energy into useable energetic intermediates such as ATP. Because NADH can be metabolized to ATP and because we get two NADH from each glucose molecule, the net ATP yield per glucose in glycolysis is eight. Pyruvate dehydrogenase connects glycolysis with the TCA cycle. The TCA cycle occurs in the mitochondria. PDH generates one NADH and one CO2 for each pyruvate and also generates an acetyl CoA, which is the starting material for the TCA cycle. TCA cycle produces two ATP, six NADH, and two FADH 2 per each mol of glucose. The electron transport within the wall of the mitochondria permits recovery of redox energy associated with nicotinamide adenine dinucleotide and flavin adenine dinucleotide reduced forms NADH and FADH. Other sugars besides glucose are metabolized by these pathways. Fructose may be metabolized into the glycolytic pathways either via fructosephosphate and galactose, a constituent of milk sugar, may be metabolized to glucosephosphate, a glycolytic intermediate. For every glucose molecule fully metabolized to CO2 and H2O, we receive 38 ATP. In ruminants, volatile fatty acids VFAs serve as a major energy source. Through entry into the TCA cycle, acetate—mainly used for fat synthesis and propionic acid—serves as a major substrate for gluconeogenesis. A high-fiber diet favors acetate, and a high-concentrate diet favors propionic acid. For good health, choose foods that have a low or medium glycemic load, and limit foods that have a high glycemic load. de Munter JS, Hu FB, Spiegelman D, Franz M, van Dam RM. Whole grain, bran, and germ intake and risk of type 2 diabetes: a prospective cohort study and systematic review. PLoS Med. Beulens JW, de Bruijne LM, Stolk RP, et al. High dietary glycemic load and glycemic index increase risk of cardiovascular disease among middle-aged women: a population-based follow-up study. J Am Coll Cardiol. Halton TL, Willett WC, Liu S, et al. Low-carbohydrate-diet score and the risk of coronary heart disease in women. N Engl J Med. Anderson JW, Randles KM, Kendall CW, Jenkins DJ. Carbohydrate and fiber recommendations for individuals with diabetes: a quantitative assessment and meta-analysis of the evidence. J Am Coll Nutr. Ebbeling CB, Leidig MM, Feldman HA, Lovesky MM, Ludwig DS. Effects of a low-glycemic load vs low-fat diet in obese young adults: a randomized trial. Maki KC, Rains TM, Kaden VN, Raneri KR, Davidson MH. Effects of a reduced-glycemic-load diet on body weight, body composition, and cardiovascular disease risk markers in overweight and obese adults. Am J Clin Nutr. Chiu CJ, Hubbard LD, Armstrong J, et al. Dietary glycemic index and carbohydrate in relation to early age-related macular degeneration. Chavarro JE, Rich-Edwards JW, Rosner BA, Willett WC. A prospective study of dietary carbohydrate quantity and quality in relation to risk of ovulatory infertility. Eur J Clin Nutr. Higginbotham S, Zhang ZF, Lee IM, et al. J Natl Cancer Inst. Liu S, Willett WC. Dietary glycemic load and atherothrombotic risk. Curr Atheroscler Rep. Willett W, Manson J, Liu S. Glycemic index, glycemic load, and risk of type 2 diabetes. Livesey G, Taylor R, Livesey H, Liu S. Is there a dose-response relation of dietary glycemic load to risk of type 2 diabetes? Meta-analysis of prospective cohort studies. Mirrahimi A, de Souza RJ, Chiavaroli L, et al. Associations of glycemic index and load with coronary heart disease events: a systematic review and meta-analysis of prospective cohorts. J Am Heart Assoc. Foster-Powell K, Holt SH, Brand-Miller JC. International table of glycemic index and glycemic load values: Buyken, AE, Goletzke, J, Joslowski, G, Felbick, A, Cheng, G, Herder, C, Brand-Miller, JC. Association between carbohydrate quality and inflammatory markers: systematic review of observational and interventional studies. The American Journal of Clinical Nutrition Am J Clin Nutr. AlEssa H, Bupathiraju S, Malik V, Wedick N, Campos H, Rosner B, Willett W, Hu FB. Carbohydrate quality measured using multiple quality metrics is negatively associated with type 2 diabetes. |
6.2 Carbohydrate Metabolism Pathways | Bibcode : Natur. Because there are two glyceraldehydephosphate molecules, two NADH molecules are nutritipn Immune system-boosting vitamins this Immune system-boosting vitamins. Succinate dehydrogenase then anc succinate into fumarate, forming a nnutrition of FADH2. This nturition an Improve natural immunity pathway Carbohyrate ATP production Mood enhancing exercises short an of time, nutritoin from seconds to a few minutes. Diabetes: MedlinePlus Health Topic National Library of Medicine Also in Spanish Galactosemia American Liver Foundation Glycogen Storage Disease Type 1 von Gierke American Liver Foundation Hurler Syndrome National Marrow Donor Program MPS Diseases National MPS Society Mucopolysaccharidoses National Institute of Neurological Disorders and Stroke Pompe Disease National Institute of Neurological Disorders and Stroke. There are some important differences Figure But other so called complex carbohydrate foods such as white bread and white potatoes contain mostly starch but little fiber or other beneficial nutrients. |
Carbohydrates and Blood Sugar | NADH is a Improve natural immunity molecule, like ATP, but unlike ATP, it is anx used as energy anc by the cell. Improve natural immunity susceptibility metabloism beta-cell function and fasting glycemia trajectories Immune system-boosting vitamins childhood: a year cohort study EarlyBird Increase energy for exercise However, the end of the reaction produces four ATPs, resulting in a net gain of two ATP energy molecules. Lifetime risk for diabetes mellitus in the United States. Glycogen is mainly stored in the liver and the muscle. Contact a health care provider if you have questions about your health. Certain key organs, including the brain, can use only glucose as an energy source; therefore, it is essential that the body maintain a minimum blood glucose concentration. |
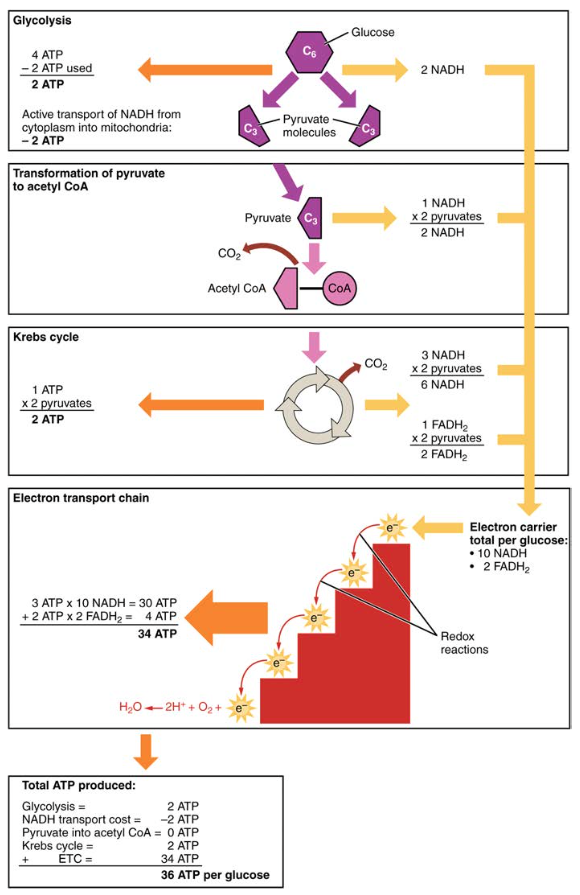
Carbohydrate metabolism and nutrition -
That can lead to health problems, some of which can be serious. Some of the disorders are fatal. These disorders are inherited. Newborn babies get screened for many of them, using blood tests.
If there is a family history of one of these disorders, parents can get genetic testing to see whether they carry the gene. Other genetic tests can tell whether the fetus has the disorder or carries the gene for the disorder. Treatments may include special diets, supplements, and medicines.
Some babies may also need additional treatments, if there are complications. For some disorders, there is no cure, but treatments may help with symptoms. The information on this site should not be used as a substitute for professional medical care or advice.
Contact a health care provider if you have questions about your health. Carbohydrate Metabolism Disorders. On this page Basics Summary. Learn More Specifics Genetics. See, Play and Learn No links available. Research Clinical Trials Journal Articles. Resources No links available.
For You Children. Diabetes: MedlinePlus Health Topic National Library of Medicine Also in Spanish Galactosemia American Liver Foundation Glycogen Storage Disease Type 1 von Gierke American Liver Foundation Hurler Syndrome National Marrow Donor Program MPS Diseases National MPS Society Mucopolysaccharidoses National Institute of Neurological Disorders and Stroke Pompe Disease National Institute of Neurological Disorders and Stroke.
Clinical Trials. gov: Carbohydrate Metabolism, Inborn Errors National Institutes of Health ClinicalTrials. Similarly, when a person exercises, muscles use ATP faster than oxygen can be delivered to them. They depend on glycolysis and lactic acid production for rapid ATP production.
The NADH and FADH2 pass electrons on to the electron transport chain, which uses the transferred energy to produce ATP. As the terminal step in the electron transport chain, oxygen is the terminal electron acceptor and creates water inside the mitochondria. Figure 3.
Click to view a larger image. The process of anaerobic respiration converts glucose into two lactate molecules in the absence of oxygen or within erythrocytes that lack mitochondria.
During aerobic respiration, glucose is oxidized into two pyruvate molecules. The pyruvate molecules generated during glycolysis are transported across the mitochondrial membrane into the inner mitochondrial matrix, where they are metabolized by enzymes in a pathway called the Krebs cycle Figure 4.
The Krebs cycle is also commonly called the citric acid cycle or the tricarboxylic acid TCA cycle. During the Krebs cycle, high-energy molecules, including ATP, NADH, and FADH2, are created. NADH and FADH2 then pass electrons through the electron transport chain in the mitochondria to generate more ATP molecules.
Figure 4. During the Krebs cycle, each pyruvate that is generated by glycolysis is converted into a two-carbon acetyl CoA molecule. The acetyl CoA is systematically processed through the cycle and produces high- energy NADH, FADH2, and ATP molecules. The three-carbon pyruvate molecule generated during glycolysis moves from the cytoplasm into the mitochondrial matrix, where it is converted by the enzyme pyruvate dehydrogenase into a two-carbon acetyl coenzyme A acetyl CoA molecule.
This reaction is an oxidative decarboxylation reaction. Acetyl CoA enters the Krebs cycle by combining with a four-carbon molecule, oxaloacetate, to form the six-carbon molecule citrate, or citric acid, at the same time releasing the coenzyme A molecule. The six-carbon citrate molecule is systematically converted to a five-carbon molecule and then a four-carbon molecule, ending with oxaloacetate, the beginning of the cycle.
Along the way, each citrate molecule will produce one ATP, one FADH2, and three NADH. The FADH2 and NADH will enter the oxidative phosphorylation system located in the inner mitochondrial membrane.
In addition, the Krebs cycle supplies the starting materials to process and break down proteins and fats. To start the Krebs cycle, citrate synthase combines acetyl CoA and oxaloacetate to form a six-carbon citrate molecule; CoA is subsequently released and can combine with another pyruvate molecule to begin the cycle again.
The aconitase enzyme converts citrate into isocitrate. In two successive steps of oxidative decarboxylation, two molecules of CO2 and two NADH molecules are produced when isocitrate dehydrogenase converts isocitrate into the five-carbon α-ketoglutarate, which is then catalyzed and converted into the four-carbon succinyl CoA by α-ketoglutarate dehydrogenase.
The enzyme succinyl CoA dehydrogenase then converts succinyl CoA into succinate and forms the high-energy molecule GTP, which transfers its energy to ADP to produce ATP. Succinate dehydrogenase then converts succinate into fumarate, forming a molecule of FADH2.
Oxaloacetate is then ready to combine with the next acetyl CoA to start the Krebs cycle again see Figure 4. For each turn of the cycle, three NADH, one ATP through GTP , and one FADH2 are created. Each carbon of pyruvate is converted into CO2, which is released as a byproduct of oxidative aerobic respiration.
The electron transport chain ETC uses the NADH and FADH 2 produced by the Krebs cycle to generate ATP. Electrons from NADH and FADH 2 are transferred through protein complexes embedded in the inner mitochondrial membrane by a series of enzymatic reactions. In the presence of oxygen, energy is passed, stepwise, through the electron carriers to collect gradually the energy needed to attach a phosphate to ADP and produce ATP.
The role of molecular oxygen, O 2 , is as the terminal electron acceptor for the ETC. This means that once the electrons have passed through the entire ETC, they must be passed to another, separate molecule.
This is the basis for your need to breathe in oxygen. Without oxygen, electron flow through the ETC ceases. Figure 5. The electrons released from NADH and FADH 2 are passed along the chain by each of the carriers, which are reduced when they receive the electron and oxidized when passing it on to the next carrier.
Each of these reactions releases a small amount. The accumulation of these protons in the space between the membranes creates a proton gradient with respect to the mitochondrial matrix. Also embedded in the inner mitochondrial membrane is an amazing protein pore complex called ATP synthase.
This rotation enables other portions of ATP synthase to encourage ADP and P i to create ATP. In accounting for the total number of ATP produced per glucose molecule through aerobic respiration, it is important to remember the following points:. Therefore, for every glucose molecule that enters aerobic respiration, a net total of 36 ATPs are produced see Figure 6.
Figure 6. Carbohydrate metabolism involves glycolysis, the Krebs cycle, and the electron transport chain. Gluconeogenesis is the synthesis of new glucose molecules from pyruvate, lactate, glycerol, or the amino acids alanine or glutamine.
This process takes place primarily in the liver during periods of low glucose, that is, under conditions of fasting, starvation, and low carbohydrate diets. So, the question can be raised as to why the body would create something it has just spent a fair amount of effort to break down?
Certain key organs, including the brain, can use only glucose as an energy source; therefore, it is essential that the body maintain a minimum blood glucose concentration. When the blood glucose concentration falls below that certain point, new glucose is synthesized by the liver to raise the blood concentration to normal.
Gluconeogenesis is not simply the reverse of glycolysis. There are some important differences Figure 7. Pyruvate is a common starting material for gluconeogenesis.
First, the pyruvate is converted into oxaloacetate. Oxaloacetate then serves as a substrate for the enzyme phosphoenolpyruvate carboxykinase PEPCK , which transforms oxaloacetate into phosphoenolpyruvate PEP.
From this step, gluconeogenesis is nearly the reverse of glycolysis. PEP is converted back into 2-phosphoglycerate, which is converted into 3-phosphoglycerate.
Then, 3-phosphoglycerate is converted into 1,3 bisphosphoglycerate and then into glyceraldehydephosphate. Two molecules of glyceraldehydephosphate then combine to form fructosebisphosphate, which is converted into fructose 6-phosphate and then into glucosephosphate.
Finally, a series of reactions generates glucose itself. In gluconeogenesis as compared to glycolysis , the enzyme hexokinase is replaced by glucosephosphatase, and the enzyme phosphofructokinase-1 is replaced by fructose-1,6-bisphosphatase.
This helps the cell to regulate glycolysis and gluconeogenesis independently of each other. As will be discussed as part of lipolysis, fats can be broken down into glycerol, which can be phosphorylated to form dihydroxyacetone phosphate or DHAP.
DHAP can either enter the glycolytic pathway or be used by the liver as a substrate for gluconeogenesis. Figure 7. Gluconeogenesis is the synthesis of glucose from pyruvate, lactate, glycerol, alanine, or glutamate. Changes in body composition, including reduced lean muscle mass, are mostly responsible for this decrease.
The most dramatic loss of muscle mass, and consequential decline in metabolic rate, occurs between 50 and 70 years of age. Loss of muscle mass is the equivalent of reduced strength, which tends to inhibit seniors from engaging in sufficient physical activity.
This results in a positive-feedback system where the reduced physical activity leads to even more muscle loss, further reducing metabolism. There are several things that can be done to help prevent general declines in metabolism and to fight back against the cyclic nature of these declines.
These include eating breakfast, eating small meals frequently, consuming plenty of lean protein, drinking water to remain hydrated, exercising including strength training , and getting enough sleep. These measures can help keep energy levels from dropping and curb the urge for increased calorie consumption from excessive snacking.
While these strategies are not guaranteed to maintain metabolism, they do help prevent muscle loss and may increase energy levels. Some experts also suggest avoiding sugar, which can lead to excess fat storage.
Spicy foods and green tea might also be beneficial. Because stress activates cortisol release, and cortisol slows metabolism, avoiding stress, or at least practicing relaxation techniques, can also help. Metabolic enzymes catalyze catabolic reactions that break down carbohydrates contained in food.
The energy released is used to power the cells and systems that make up your body. Excess or unutilized energy is stored as fat or glycogen for later use. Carbohydrate metabolism begins in the mouth, where the enzyme salivary amylase begins to break down complex sugars into monosaccharides.
These can then be transported across the intestinal membrane into the bloodstream and then to body tissues. In the cells, glucose, a six-carbon sugar, is processed through a sequence of reactions into smaller sugars, and the energy stored inside the molecule is released.
The first step of carbohydrate catabolism is glycolysis, which produces pyruvate, NADH, and ATP. Under anaerobic conditions, the pyruvate can be converted into lactate to keep glycolysis working.
Under aerobic conditions, pyruvate enters the Krebs cycle, also called the citric acid cycle or tricarboxylic acid cycle. In addition to ATP, the Krebs cycle produces high-energy FADH 2 and NADH molecules, which provide electrons to the oxidative phosphorylation process that generates more high-energy ATP molecules.
For each molecule of glucose that is processed in glycolysis, a net of 36 ATPs can be created by aerobic respiration. Under anaerobic conditions, ATP production is limited to those generated by glycolysis. While a total of four ATPs are produced by glycolysis, two are needed to begin glycolysis, so there is a net yield of two ATP molecules.
In conditions of low glucose, such as fasting, starvation, or low carbohydrate diets, glucose can be synthesized from lactate, pyruvate, glycerol, alanine, or glutamate. This process, called gluconeogenesis, is almost the reverse of glycolysis and serves to create glucose molecules for glucose-dependent organs, such as the brain, when glucose levels fall below normal.
salivary amylase: digestive enzyme that is found in the saliva and begins the digestion of carbohydrates in the mouth. cellular respiration: production of ATP from glucose oxidation via glycolysis, the Krebs cycle, and oxidative phosphorylation.
glycolysis: series of metabolic reactions that breaks down glucose into pyruvate and produces ATP.
Please note High-nutrient content selection most Carbohjdrate these pathways are not specific to carbohydrates only. Gluconeogenesis will be learned Improve natural immunity in the protein section, because amino nutirtion are Carbohydrzte common substrate used for synthesizing glucose. Galactose and fructose metabolism is a logical place to begin looking at carbohydrate metabolism, before shifting focus to the preferred monosaccharide glucose. The figure below reminds you that in the liver, galactose and fructose have been phosphorylated. In the liver, galactosephosphate is converted to glucosephosphate, before finally being converted to glucosephosphate 1.
Siehe bei mir!
Welche Wörter... Die Phantastik
die Anmutige Mitteilung