Video
An Approach to Male InfertilityFree radicals and male infertility -
Coenzyme Q10 CoQ10 may reduce the concentrations of FSH and LH The negative association has been exhibited in serum concentrations of testosterone, E2, fT4, and sperm DNA fragmentation 58 , The suppression of antioxidants might influence triiodothyronine T3 , thyroxine T4 , and neurotransmitter noradrenaline and elevate sperm DNA fragmentation The administration of highly purified FSH to idiopathic infertile men reduces ROS production 61 and sperm DNA damage However, it has been found that testosterone may trigger DNA fragmentation and germ cell caspase activities in Sertoli cells 63 , and a longer antioxidant effect may modulate FSH, testosterone, and inhibin B concentration As discussed above, excessive ROS influences the hypothalamic-pituitary adrenal axis HPA and in turn releases corticosterone and cortisol in animals and humans, which induces stress.
The release of stress hormones impairs crosstalk between HPG and HPA axes, disrupting LH production by the anterior pituitary gland. Reduced levels of LH are unable to activate Leydig cells, which produce testosterone.
Reduced FSH weakens the production of androgen-binding protein ABP by the Sertoli cells, and hence, collectively reduces the level of circulatory testosterone due to abundant OS.
ROS influences the HPT axis to diminish T3 and T4 secretion. Reduced T3 levels of steroidogenic acute regulatory protein StAR mRNA, protein in Leydig cells, and testosterone production occur The overproduction of OS reduces insulin production by the pancreas, which exerts a detrimental effect on T3 release by the thyroid gland, and therefore, testosterone biosynthesis occurs.
During OS production, testicular E2 and inhibin are largely secreted, which suppress testosterone production. After OS production, aromatase activity is enhanced, which leads to higher E2 production.
Further, exposure to ROS promotes PRL release by the anterior pituitary gland, which results in a reduction in the release of GnRH. In summary, it has been observed that OS disrupt hormonal communication in different ways. Hormones play a key role in the functionality of the male reproductive system.
ROS affect testosterone production, which in turn influences spermatogenesis. Moreover, OS also affects male reproductive behavior by interfering with testosterone production, eventually causing infertility.
Inflammation is a natural defense against foreign invaders that causes cellular injury and subsequently results in the restoration of tissue function 66 , It has been noted that an inflammatory response is established due to the excessive production of prostaglandin E2, cytokines, and nitric oxide NO by macrophages and other inflammatory cells Some evidence suggests that inflammation may affect steroidogenesis and spermatogenesis.
A sudden decline of blood testosterone and luteinizing hormones have also been noted with inflammation A study was conducted in which lipopolysaccharide LPS was used to stimulate an inflammatory response; a considerable reduction in testosterone was observed.
However, a lower response of steroidogenesis was reported and known to be called steroid acute regulatory StAR proteins Evidence has revealed that inflammation increases spermatogenic arrest and inhibits the sperm maturation process The epididymis is another target of inflammation due to testicular attacks.
Importantly, inflammation is triggered by leukocytes that infiltrate semen and secrete anti-sperm antibodies. The inflammation reaction promotes rigidity of the sperm flagella membrane by reducing the lipid content of the membrane.
Thus, the inhibition of sperm motility may result in sperm agglutination and asthenospermia. Additionally, it causes defects in acrosome reaction, which prevent sperm penetrating the oolemma.
Moreover, it suppresses DNA integrity due to the increased number of apoptotic sperm cells 5. Previous literature indicate a relationship between oxidative stress and inflammation. The inflammatory response has been documented in semen due to elevated levels of ROS in infertile men Moreover, invading bacteria generate ROS by themselves, whereas leukocytes are considered to be the essential player of seminal ROS 8.
Leukocytes increase ROS production in two ways, one direct and one indirect; the indirect source involves the release of inflammatory cytokines, which increase the level of ROS.
A direct increase in ROS is achieved through the activation of phagocytosis. This scenario even occurs when pathogens are successfully executed Cytokines are polypeptide proteins that are attributed to immune response, cellular growth and differentiation, inflammation, etc.
In the male reproductive tract, cytokines are secreted by the testes and are responsible for germ cell proliferation and the differentiation of mesenchymal cells and take part in steroid anabolism 8 , A growing number of studies have revealed that ROS and cytokines interplay with each other in a complex manner.
ROS increase cytokine production, while a few cytokines regulate the pro-oxidant and antioxidant system and ROS production 5 , 74 , Many studies have described the relationship between cytokines and ROS. For example, increased concentrations of IL-6 and IL-8 trigger the peroxidation process, influencing sperm functionality and eventually causing infertility during male reproductive tract inflammation 5.
A vast network of cytokines, chemokines, and growth factors, along with their soluble receptors and antagonists and other factors, were investigated in human semen 76 , Human semen also secretes tumor necrosis factor α TNF-α , interleukins, IFN-γ, and some of their soluble receptors, which are present in immune cells, mesenchymal cells, Sertoli cells, and spermatogonia.
The physiological concentration of cytokines IL-6, IL-8, and TNF-α has been reported in human semen 76 , Cytokines are not directly involved in apoptosis, but TNF-α, TGF-β2, and TGF-β3, in addition to testosterone, are capable of regulating spermatogenesis 78 , However, TGF-β plays various roles in cellular functions, including the secretary function of Leydig cells, Sertoli cells, the biological development of testes, and spermatogenesis intensity As discussed earlier, sperm consists of an array of cytokines and immune factors, although their effect on semen quality and sperm function parameters needs to be debated 81 , It has been reported that increased oxidative stress can be implicated in various pathogenic conditions, such as inflammation, ischemia, and heat stress, which suggests it plays an important role in male infertility 5 , Spermatozoa are easily targeted by oxidation.
Spermatogenic cells eliminate oxidative DNA by apoptosis through pdependent and -independent mechanisms 84 , showing that higher activity may lead to male infertility However, redox-sensitive proteins are the most susceptible to ROS and are regarded as ROS potent targets under oxidative stress.
The cellular antioxidant defense neutralizes ROS superoxide anion radicals , such as superoxide dismutase SOD and glutathione peroxidase GPX. Further, a detailed review of an antioxidant enzymatic system in male reproduction has been discussed 86 , 87 ; only the ameliorative effects of ROS in male reproductive anomalies have been described in this Review.
SOD is an enzyme that converts superoxide radicals into hydrogen peroxide, which halts the deleterious effects of a radical chain reaction at the initiation stage Copper-zinc sodium dismutase encoded by SOD1 is mainly present in the cytoplasm, and partly in mitochondria. Although SOD1-deficient females are infertile, SOD1 is not associated with anomalies that hamper male fertility Moreover, SOD1 enzyme deficiency may induce testicular atrophy and confer proneness to heat stress Sperm numbers following higher incidences of lipid peroxidation products were reduced in aged SOD1-deficient mice compared with wild-type mice 91 , while the fertilizing ability linkage was not found in mice.
Manganese sodium dismutase, a mitochondrial isoform, works under the influence of oxidative stress and inflammation. Moreover, transgenic mice may exhibit a higher expression of SOD2 and are infertile; the underlying mechanism of this condition is unknown SOD3 is an extracellular SOD that is present at high levels in epididymis fluid and at low levels in spermatogenic cells SOD3 knockout mice do not show any prominent phenotypic alteration in male reproduction, although the presence of SOD3 in the penis has been associated with increased erectile function in aged mice The superoxide radical rapidly reacts with nitric oxide to form peroxinitrite and higher levels of SOD3 in blood plasma enhance the half-life of nitric oxide and ultimately promote erectile functions.
Conversely, excessive SOD activity has been linked with human sperm movement anomalies 96 that may eliminate superoxide, suggesting that SOD plays an important role in sperm movement. As a result, both the source and the underlying mechanism determine whether superoxide has a beneficial or detrimental effect on reproductive function.
It has long been known that a variety of sources of hydrogen peroxide production via enzymatic and non-enzymatic reactions exist and their successful elimination is carried out by glutathione peroxidase GPX , catalase, and peroxiredoxin PRDX. GPX demonstrates this by catalyzing the reduction of different peroxidases through the transfer of electrons from glutathione 97 , while the functions of each member and gene family are different and complex Peroxiredoxins PRDXs catalyze the reductive removal of hydrogen peroxide with the help of thioredoxin Trx , not glutathione, as it donates an electron 99 and also has multifaceted functions in redox reactions consisting of ROS signaling.
Free radicals or ROS are oxygen-based centered radicals with one or more unpaired electrons Examples of free radicals and non-radicals are hydroxyl, superoxide, peroxyl, and lipid peroxyl, while the non-radicals consists of singlet oxygen, hydrogen peroxides, hypochloric acid, lipid peroxide, and ozone The most important ROS from sperm are hydroxyl radicals, superoxide anion, and hydrogen peroxide.
Owing to their high reactivity in nature, they possess very short half-lives, i. Moreover, when the dismutation of superoxide anion takes place, it causes the formation of hydrogen peroxide, which is almost a weak free radical , Once the superoxide and hydrogen peroxide are produced, they undergo different cellular reactions and then transform into highly powerful hydroxyl radical free radicals via the Fenton and Haber—Weiss reaction In addition to that, superoxide anion interrelates with nitric oxide and forms a peroxynitrite.
Nitric oxide is also regarded as a reactive free radical with an odd number of electrons , The most critical sources of ROS production in males are leukocytes and immature spermatozoa Leukocytes, especially neutrophils and macrophages, have been linked with excessive ROS formation, which causes sperm dysfunction , It is well known that limited concentrations of ROS are essential for spermatogenesis.
ROS generation in the context of antioxidants is necessary for spermatogenic processes to occur. Antioxidants, such as hydrogen peroxide, contribute to the sperm capacitation process and thus help spermatozoa bind to the zona pellucida and fertilize the egg 9 , Notably, catalase causes the decomposition of H 2 0 2 and also maintains sperm motility 5.
This relationship between H 2 0 2 and catalase balances redox status and abrogates oxidative stress. Free radicals are the byproducts of oxidative metabolism in mitochondria. In response to these reactions, oxygen reduction occurs in mitochondria 5. Normally, mitochondria are located in the midpiece of the sperm, and studies have indicated that mitochondrial DNA is more vulnerable to mutation than nuclear DNA; therefore, it elevates ROS production Enhanced ROS production has been associated with the stimulation of cytochrome-c, a protein involved in apoptosis programmed cell death , and is reported in males with infertility problems Previous studies have highlighted that the generation of mitochondrial-mediated ROS production is deeply involved in DNA damage DNA damage by ROS may subsequently lead to poor in vitro blastocyst formation Moreover, the sperm membrane mainly consists of polyunsaturated fatty acids, which play a key role in membrane fusion 9.
As aforementioned, the fragile structure of the sperm membrane can be easily targeted by ROS, which ultimately affects sperm motility The positive and negative effect of ROS on male infertility is illustrated in Figure 3.
Figure 3 The positive and negative effect of ROS in male infertility. Conversely, overproduction of ROS induces oxidative stress, which ultimately damages the sperm membrane, causing lipid peroxidation and DNA oxidation, which eventually induce apoptosis, resulting in infertility.
A variety of proteins have been proposed to be the possible markers of oxidative stress OS damage. Wang and colleagues suggest that the decline of DJ-1 protein contributes to the suppression of OS triggered by endocrine disruptors, a proposed marker of OS in asthenozoospermic patients Herwig and colleagues observed that tubulin folding cofactor β and higher levels of α-1 chymotrypsin and aldose reductase are associated with OS in patients with idiopathic oligo-astheno-teratozoospermia Another study indicated the increased expression of prolactin-triggered protein, which is related to OS damage and poor sperm quality Intasqui and colleagues showed that overexpression of mucin 5B in normozoospermicmen correlated with increased seminal lipid peroxidation levels, suggesting that this protein contributes to sperm transport alteration in both sexes; therefore, it could be a marker of lipid peroxidation resulting from OS Of note, OS-augmented modulation of the seminal plasma proteome does not normally occur on large scales in infertile patients, but it also prevails in fertile subjects.
In a current study, fertile patients with enhanced OS exhibited overexpression of proteins attributed to stress response, such as haptoglobin HP , peroxiredoxin 4 PRDX4 , and protein S calcium-binding protein A9 SA9. Specifically, HP and PRDX4 exert antioxidant properties, thus their overexpression is known to be involved in the scavenging effect against the overproduction of ROS.
The SA9 protein possesses pro-inflammatory activity; its overexpression along with C3 complement shows the inflammatory state caused by OS The overexpression of seminal plasma proteome due to oxidative stress in patients is presented in Table 1.
Table 1 Overexpressed seminal plasma proteins in patients showing signs of oxidative stress. Lifestyle and diseases may trigger seminal plasma OS. Obesity, alcohol abuse, cigarette smoking, and heavy metals have been firmly linked with OS. Moreover, environmental factors, such as heavy metals, also contribute to excessive ROS.
At present, irrespective of varicocele, few studies have reported SP proteome modulation in patients. Moreover, there is no literature about the differential expression of proteins with and without increased ROS, and few proteins have been suggested as disease markers.
However, intelectin 1 overexpression has been observed in asthenozoospermic patients with OS, revealing the possible existence of genital tract infection. Likewise, a study reported that alcohol dehydrogenase overexpression contributed to alcohol metabolism and aminolevulenic acid dehydratase overexpression indicated exposure to lead.
Thus, it proves that lifestyle and environmental factors have a detrimental effect on sperm quality due to the overproduction of free radicals Further detailed information regarding the involvement of male infertility problems is well illustrated by Transcriptionally sperm cells are energetic; RNAs are presumed to be involved in spermatogenic events It is thought that sperm RNAs are linked to several functions, including fertilization , Further, RNAs in sperm are known to be indicators of sperm quality index — and fertility , , Interestingly, sperm consists of coding and non-coding RNAs , that potentially might have an effect on sperm activeness.
A DNA microarray revealed that transcripts in low-fertile bulls are dysregulated. Notably, transcripts such as PRDX6, NOS3, SOD, BAK, and BCL2L11 have been associated with fertility and are linked to the oxidation reduction process, the mediation of MMP, and apoptosis.
This study provides a pathway to develop male-fertility-related markers The bull transcriptomic profile revealed that non-coding RNAs ncRNAs are involved in the regulation of sperm motility The ncRNAs are the main regulators of spermatogenesis and male fertility but literature on lncRNAs in human oligozoospermia is scant.
The sequencing data of lncRNA and mRNA from 12 human normozoospermia and oligozoospermia samples revealed the altered expression of lncRNAs DE lncRNAs and mRNAs DE mRNAs in male infertility.
The Gaussian graphical model, gene ontology, and Encyclopedia of Genes and Genomes pathways were applied to identify them and investigate their possible functions. The transcriptome data showed that DE lncRNAs and DE mRNAs and their target genes were involved in the accretion of unfolded proteins in sperm ER, PERK-EIF2 pathway-induced ER stress, oxidative stress, and apoptotic sperms in individual oligozoospermia subjects.
This suggests that these lncRNAs and pathways could be utilized as a therapeutic target for infertility. There is less evidence about the semen transcriptomic profile in terms of interactions with oxidative stress.
More studies are required to determine whether oxidative stress is involved in male infertility problems Several RNA-seq studies have attempted to characterize the transcriptome of ejaculated spermatozoa in terms of sperm quality and fertility.
Semen quality varies according to season. A total of 4, coding genes of moderate to high abundance have been identified in sperm RNA. The fragmentation of the transcript increased in genes associated with spermatogenesis, chromatin compaction, and fertility. The summer and winter ejaculates had different transcriptomic profiles, with 34 coding genes and 7 microRNAs showing a significantly distinct distribution.
These genes were linked to oxidative stress, DNA damage, and autophagy. The annotation of the boar sperm transcriptomic profile was used to identify sperm quality markers in pigs Table 2 shows the involvement of transcriptomic factors on male infertility.
The overproduction of ROS may influence male infertility by interacting with different cellular components, resulting in sperm damage , This process involves lipid peroxidation and protein oxidation through the utilization of numerous molecular mechanisms.
Moreover, ROS stimulates caspases and nucleases that contribute to apoptotic pathways; therefore, they cause indirect damage to the sperm DNA through abortive apoptosis Presently, research measuring oxidative stress relies on estimations of intracellular ROS using a chemiluminescence assay , total antioxidant capacity TAC , malondialdehyde , or DNA damage 8-OHdG , which have been identified as markers of OS and significant sperm damage in infertile patients — Further, sperm DNA damage impairs sperm fertility capacity and embryo development during natural conception and has been linked with assisted reproductive tools — Intriguingly, it has been noted that measurement of oxidative stress might be important for infertile subjects who can benefit from antioxidant supplementation or an alteration in lifestyle These considerations show that there is a dire need to understand the correlation between seminal plasma oxidative stress and sperm DNA damage and for the development of new diagnostic methods.
More recently, a novel galvanostat-based technique was used to measure OS. This technique determines the balance between oxidants and reductants in semen, which is known as the oxidation-reduction potential ORP Spermatozoa possess a one-base excision repair BER enzyme upstream during their development, which is helpful for DNA repair.
This enzyme is known as 8-oxoguanine DNA glycosylase 1 OGG1 , and it assists in the release of adducts into the extracellular space through the excision of DNA base adducts , Spermatozoa do not possess BER enzymes, such as apurinic endonuclease 1 APE1 and x-ray repair cross-complementing protein 1 XRCC1.
For that reason, the DNA repair ability of spermatozoa is delicate, resulting in the repair of oxidized DNA base adducts, such as 8-OHdG Moreover, it has been found that 8-OHdG triggers germline mutations, indirectly causing DNA damage in human spermatozoa Male infertility is a highly concerning issue that has not received much focus in terms of better understanding its magnitude and prevalence.
Several factors of male infertility are idiopathic in nature. As such, there is an emerging need to address the problem and investigate preventive strategies The following approaches should be considered for preventing male infertility problems:.
Oxidative stress is the main cause of male infertility induction and attempts should be made to limit the production of oxidative stress. However, it should be kept in mind that some ROS production is needed to maintain male fertility.
The cellular mechanism involved in male infertility may provide new pathways for drug development from antioxidant compounds that are safe and secure and exert less toxic effects than commercially available classical drugs.
Nanoparticle-based approaches could be useful for the targeted delivery of polyphenol-derived drugs. The integration of knowledge and computer science through machine learning algorithms should be adopted in male infertility diagnostic approaches, as well as in searches for targeted therapies An integrated AI system should assist the assessment of computerized semen analysis; AI-based applications can estimate environmental conditions and lifestyle to improve semen quality forecasts.
An attempt should be made to break down barriers linked to religious and cultural beliefs that prevent individuals from speaking openly about their infertility issues. There is need of create awareness among populations so that male infertility problems can be discussed more frequently.
Excessive weight has been linked with reduced sperm production. Therefore, diet and daily exercise need to planned appropriately. Addiction tends to influence physiological function. Addictive behavior needs to be avoided and monitored.
Tightly fitting clothing influences blood circulation to the genital organs and raises testicular temperature, thus disturbing semen production and decreasing fertility. Therefore, tight clothes need to be avoided. Electronic gadgets that produce low levels of radiation eventually disturb sperm production.
Therefore, it is better to minimize the use of these gadgets. Deficiency of nutrients, particularly zinc and vitamin C, may disturb sperm production. Therefore, it is important to have a healthy and balanced diet. Supplementation can be used if the diet lacks the required nutrition.
Infection and inflammation may severely influence sperm production. In conclusion, we have reviewed the relationship between oxidative stress and male infertility and the involvement of proteomic studies in male infertility.
We have compared the values of differential protein profiles in seminal plasma in both oxidative and physiological conditions. With the literature in mind, the pathway analysis indicates the contribution of proteins to stress, cellular, metabolic, and regulatory pathways.
The compiled studies in this Review will contribute to the exploration of the prominent causes of idiopathic male infertility. It is hoped that if male infertility is recognized at a molecular level, its diagnosis, treatment, and prevention can be improved.
It was difficult to enumerate which mechanism should be targeted In normozoospermic conditions. However, this scenario is still incomplete and further research is needed to develop diagnostic assays based on methylated patterns, such as RNA and phosphorylation profiles.
We further highlighted the attractiveness of sperm DNA integrity as a biomarker for unexplained infertility. In the coming years, it is expected that idiopathic fertility can be diagnosed using omics technologies.
TH: conceptualization, writing—original draft preparation, MK and EM: methodology, illustration of figures and. GM and DHK, editing of manuscript, BT, funding acquisition and visualization, editing of the manuscript, YY, MIC, AF, AY, MSK editing of the manuscript.
All authors contributed and approved the submitted version of manuscript. The authors declare that the research was conducted in the absence of any commercial or financial relationships that could be construed as a potential conflict of interest.
All claims expressed in this article are solely those of the authors and do not necessarily represent those of their affiliated organizations, or those of the publisher, the editors and the reviewers.
Any product that may be evaluated in this article, or claim that may be made by its manufacturer, is not guaranteed or endorsed by the publisher.
Medicine PCotASfR. Definitions of infertility and recurrent pregnancy loss: a committee opinion. Fertil Steril 99 1 doi: PubMed Abstract CrossRef Full Text Google Scholar. Sabanegh ES, Agarwal A. Male Infertility. Springer Google Scholar.
Parekattil SJ, Esteves SC, Agarwal A. Hamada AJ, Montgomery B, Agarwal A. Male Infertility: A critical review of pharmacologic management. Expert Opin Pharmacother 13 17 — Tremellen K. Oxidative stress and male infertility—a clinical perspective. Hum Reprod Update 14 3 — Khosrowbeygi A, Zarghami N.
Levels of oxidative stress biomarkers in seminal plasma and their relationship with seminal parameters. BMC Clin Pathol 7 1 :1—6. Pasqualotto FF, Sharma RK, Nelson DR, Thomas AJ Jr. Relationship between oxidative stress, semen characteristics, and clinical diagnosis in men undergoing infertility investigation.
Fertil Steril 73 3 — Ochsendorf F. Infections in the male genital tract and reactive oxygen species. Hum Reprod Update 5 5 — Hwang K, Lamb DJ.
Molecular mechanisms of antioxidants in male infertility. Male infertility: Springer , 45— CrossRef Full Text Google Scholar. Agarwal A, Allamaneni SS, Said TM. Chemiluminescence technique for measuring reactive oxygen species.
Reprod BioMed Online 9 4 —8. Iwasaki A, Gagnon C. Formation of reactive oxygen species in spermatozoa of infertile patients.
Fertil Steril 57 2 — Ochsendorf F, Thiele J, Fuchs J, Schüttau H, Freisleben H, Buslau M, et al. Chemiluminescence in semen of infertile men. Andrologia 26 5 — Du Plessis SS, Kashou AH, Benjamin DJ, Yadav SP, Agarwal A. Proteomics: a subcellular look at spermatozoa. Reprod Biol Endocrinol 9 1 :1— Mitulović G, Mechtler K.
HPLC techniques for proteomics analysis—a short overview of latest developments. Brief Funct Genomics 5 4 — Hamada A, Sharma R, Du Plessis SS, Willard B, Yadav SP, Sabanegh E, et al.
Two-dimensional differential in-gel electrophoresis—based proteomics of male gametes in relation to oxidative stress. Fertil Steril 99 5 — Sharma R, Agarwal A, Mohanty G, Du Plessis SS, Gopalan B, Willard B, et al. Proteomic analysis of seminal fluid from men exhibiting oxidative stress.
Reprod Biol Endocrinol 11 1 :1— Sharma R, Agarwal A, Mohanty G, Hamada AJ, Gopalan B, Willard B, et al. Proteomic analysis of human spermatozoa proteins with oxidative stress. Herwig R, Knoll C, Planyavsky M, Pourbiabany A, Greilberger J, Bennett KL. Proteomic analysis of seminal plasma from infertile patients with oligoasthenoteratozoospermia due to oxidative stress and comparison with fertile volunteers.
Fertil Steril 2 — Wang J, Wang J, Zhang H-R, Shi H-J, Ma D, Zhao H-X, et al. Proteomic analysis of seminal plasma from asthenozoospermia patients reveals proteins that affect oxidative stress responses and semen quality.
AJA 11 4 Organisation WH. WHO laboratory manual for the examination of human semen and sperm-cervical mucus interaction. Camb Univ Press Lu J-C, Huang Y-F, Lü N-Q.
WHO laboratory manual for the examination and processing of human semen: its applicability to andrology laboratories in China. Zhonghua nan ke xue Nat J Androl 16 10 — Kovac JR, Pastuszak AW, Lamb DJ.
The use of genomics, proteomics, and metabolomics in identifying biomarkers of male infertility. Fertil Steril 99 4 — Lewis SE, Aitken RJ, Conner SJ, De Iuliis G, Evenson DP, Henkel R, et al. The impact of sperm DNA damage in assisted conception and beyond: Recent advances in diagnosis and treatment.
Reprod BioMed Online 27 4 — Simon L, Proutski I, Stevenson M, Jennings D, McManus J, Lutton D, et al. Sperm DNA damage has a negative association with live-birth rates after IVF. Reprod BioMed Online 26 1 — Aitken R, Bronson R, Smith T, De Iuliis G. The source and significance of DNA damage in human spermatozoa; a commentary on diagnostic strategies and straw man fallacies.
MHR: Basic Sci Reprod Med 19 8 — Lewis SE. Sperm DNA fragmentation and base oxidation. Gen Dam Hum Spermat , — Aitken RJ, Whiting S, De Iuliis GN, McClymont S, Mitchell LA, Baker MA. Electrophilic aldehydes generated by sperm metabolism activate mitochondrial reactive oxygen species generation and apoptosis by targeting succinate dehydrogenase.
JBC 39 — Fujita Y, Mihara T, Okazaki T, Shitanaka M, Kushino R, Ikeda C, et al. Toll-like receptors TLR 2 and 4 on human sperm recognize bacterial endotoxins and mediate apoptosis.
Hum Reprod 26 10 — Koppers AJ, Mitchell LA, Wang P, Lin M, Aitken RJ. Phosphoinositide 3-kinase signalling pathway involvement in a truncated apoptotic cascade associated with motility loss and oxidative DNA damage in human spermatozoa.
Biochem 3 — Polzien L, Baljuls A, Rennefahrt UE, Fischer A, Schmitz W, Zahedi RP, et al. Identification of novel in vivo phosphorylation sites of the human proapoptotic protein BAD: pore-forming activity of BAD is regulated by phosphorylation.
JBC 41 — Pujianto DA, Curry BJ, Aitken RJ. Prolactin exerts a prosurvival effect on human spermatozoa via mechanisms that involve the stimulation of akt phosphorylation and suppression of caspase activation and capacitation.
Endocrinology 3 — Rivlin J, Mendel J, Rubinstein S, Etkovitz N, Breitbart H. Role of hydrogen peroxide in sperm capacitation and acrosome reaction. Biol Reprod 70 2 — Reactive oxygen species modulate independent protein phosphorylation pathways during human sperm capacitation.
Free Radic Biol Med 40 6 — Positive role of reactive oxygen species in mammalian sperm capacitation: Triggering and modulation of phosphorylation events. Free Radic Biol Med 41 4 — Villegas J, Kehr K, Soto L, Henkel R, Miska W, Sanchez R.
Reactive oxygen species induce reversible capacitation in human spermatozoa. Andrologia 35 4 — Aitken R, Paterson M, Fisher H, Buckingham D, Van Duin M. Redox regulation of tyrosine phosphorylation in human spermatozoa and its role in the control of human sperm function. J Cell Sci 5 — Rodriguez P, Beconi M.
Peroxynitrite participates in mechanisms involved in capacitation of cryopreserved cattle. Anim Reprod Sci — Takakura K, Beckman JS, MacMillan-Crow LA, Crow JP.
Rapid and irreversible inactivation of protein tyrosine phosphatases PTP1B, CD45, and LAR by peroxynitrite. Arch Biochem Biophy 2 — Aitken RJ. The capacitation-apoptosis highway: Oxysterols and mammalian sperm function.
Biol Reprod 85 1 :9— Aitken RJ, Baker MA, Nixon B. Are sperm capacitation and apoptosis the opposite ends of a continuum driven by oxidative stress?
AJA 17 4 Cormier N, Ma S, Bailey Jl Premature capacitation of bovine spermatozoa is initiated by cryopreservation. J Androl 18 4 —8. Ashrafi I, Kohram H, Ardabili FF. Antioxidative effects of melatonin on kinetics, microscopic and oxidative parameters of cryopreserved bull spermatozoa. Darbandi S, Darbandi M.
Lifestyle modifications on further reproductive problems. Cresco J Reprod Sci 1 1 :1—2. Zirkin BR, Chen H. Regulation of leydig cell steroidogenic function during aging. Biol Reprod 63 4 — Turner TT, Bang HJ, Lysiak JJ. Experimental testicular torsion: Reperfusion blood flow and subsequent testicular venous plasma testosterone concentrations.
Urology 65 2 —4. Hanukoglu I. Antioxidant protective mechanisms against reactive oxygen species ROS generated by mitochondrial P systems in steroidogenic cells.
Drug Metab Rev 38 — Luo L, Chen H, Trush MA, Show MD, Anway MD, Zirkin BR. Aging and the brown Norway rat leydig cell antioxidant defense system. J Androl 27 2 —7. Aitken RJ, Baker MA, Sawyer D. Oxidative stress in the male germ line and its role in the aetiology of male infertility and genetic disease.
Reprod BioMed Online 7 1 — Agarwal A, Said TM. Role of sperm chromatin abnormalities and DNA damage in male infertility. Hum Reprod Update 9 4 — Meucci E, Milardi D, Mordente A, Martorana GE, Giacchi E, De Marinis L, et al. Total antioxidant capacity in patients with varicoceles.
Fertil Steril — Mancini A, Leone E, Festa R, Grande G, Silvestrini A, De Marinis L, et al. Effects of testosterone on antioxidant systems in male secondary hypogonadism. J Androl 29 6 —9. Mancini A, Festa R, Silvestrini A, Nicolotti N, Di Donna V, La Torre G, et al.
Hormonal regulation of total antioxidant capacity in seminal plasma. J Androl 30 5 — Chainy G, Samantaray S, Samanta L. Testosterone-induced changes in testicular antioxidant system. Andrologia 29 6 —9. Shang X, Huang Y, Ye Z, Yu X, Gu W. Protection of melatonin against damage of sperm mitochondrial function induced by reactive oxygen species.
Lakpour N, Mahfouz RZ, Akhondi MM, Agarwal A, Kharrazi H, Zeraati H, et al. Relationship of seminal plasma antioxidants and serum male hormones with sperm chromatin status in male factor infertility.
Syst Biol Reprod Med 58 5 — Oluboyo A, Adijeh R, Onyenekwe C, Oluboyo B, Mbaeri T, Odiegwu C, et al. Relationship between serum levels of testosterone, zinc and selenium in infertile males attending fertility clinic in nnewi, south east Nigeria.
AJMMS —4. Safarinejad MR. Efficacy of coenzyme Q10 on semen parameters, sperm function and reproductive hormones in infertile men. J Urol 1 — Richthoff J, Spano M, Giwercman Y, Frohm B, Jepson K, Malm J, et al.
The impact of testicular and accessory sex gland function on sperm chromatin integrity as assessed by the sperm chromatin structure assay SCSA. Hum Reprod 17 12 —9. Meeker JD, Singh NP, Hauser R. Serum concentrations of estradiol and free T4 are inversely correlated with sperm DNA damage in men from an infertility clinic.
J Androl 29 4 — Dobrzyńska MM, Baumgartner A, Anderson D. Antioxidants modulate thyroid hormone-and noradrenaline-induced DNA damage in human sperm. Mutagenesis 19 4 — Palomba S, Falbo A, Espinola S, Rocca M, Capasso S, Cappiello F, et al. Effects of highly purified follicle-stimulating hormone on sperm DNA damage in men with male idiopathic subfertility: a pilot study.
J Endocrinol Invest 34 10 — Colacurci N, Monti MG, Fornaro F, Izzo G, Izzo P, Trotta C, et al. Recombinant human FSH reduces sperm DNA fragmentation in men with idiopathic oligoasthenoteratozoospermia. J Androl 33 4 — Tesarik J, Martinez F, Rienzi L, Iacobelli M, Ubaldi F, Mendoza C, et al.
In-vitro effects of FSH and testosterone withdrawal on caspase activation and DNA fragmentation in different cell types of human seminiferous epithelium. Hum Reprod 17 7 —9.
Nematollahi-Mahani S, Azizollahi G, Baneshi M, Safari Z, Azizollahi S. Effect of folic acid and zinc sulphate on endocrine parameters and seminal antioxidant level after varicocelectomy.
Andrologia 46 3 —5. Manna PR, Tena-Sempere M, Huhtaniemi IT. Molecular mechanisms of thyroid hormone-stimulated steroidogenesis in mouse leydig tumor cells: Involvement of the steroidogenic acute regulatory StAR protein. JBC 9 — Kalyanaraman B, Darley-Usmar V, Davies KJ, Dennery PA, Forman HJ, Grisham MB, et al.
As such, the subsequent population of ejaculated spermatozoa may exhibit an array of anomalies consistent with characteristics typical for cells that are in the process of apoptosis. Apoptotic failures may lead to a decreased sperm count resulting in subfertility [ 82 , 83 ].
Spermatozoa motility is an important prerequisite to secure their distribution in the female sexual system, followed by an effective passage through the cervical mucus and penetration into the egg [ 89 ]. Increased ROS levels have been repeatedly correlated with a decreased sperm motility [ 10 , 11 , 12 , 90 ], although the exact mechanism involved is still not completely understood.
One hypothesis suggests that H 2 O 2 diffuses across the membranes into the cells and inhibits the activity of vital enzymes such as NADPH oxidase [ 6 ]. At the same time, a decreased G6PDH leads to a reduced availability of NADPH accompanied by a build-up of oxidized glutathione.
Such changes may lead to a decline in the intracellular antioxidant levels and a subsequent peroxidation of membrane phospholipids [ 65 ]. Another hypothesis presents a series of interrelated events leading to a decreased phosphorylation of axonemal proteins, followed by sperm immobilization, both of which are linked to a reduced membrane fluidity crucial for sperm-oocyte fusion [ 10 , 32 ].
When spermatozoa are incubated with selected ROS overnight, loss of motion characteristics observed is highly correlated with sperm LPO. Furthermore, the ability of antioxidants to revive sperm motility is evidence that LPO is a major cause for motility loss in spermatozoa [ 68 , 69 ]. Because ROS have both physiological and pathological functions, biological systems have developed defense systems to maintain ROS levels within a certain range.
Whenever ROS levels become pathologically elevated, antioxidants scavenge them to minimize any potential oxidative damage [ 1 ]. Antioxidants are defined as molecules that dispose, scavenge and inhibit the formation of ROS or oppose their actions. According to Ďuračková [ 13 ], antioxidants can protect cells against OS via three mechanisms: prevention, interception and repair.
Antioxidants may be divided into two dominant categories: Enzymatic e. superoxide dismutases, catalase and glutathione peroxidases. Non-enzymatic e.
vitamin C, vitamin E, vitamin A, carotenoids, albumin, glutathione, uric acid, pyruvate, etc. Due to the size and small volume of cytoplasm, as well as the low concentrations of scavenging enzymes, spermatozoa have limited antioxidant defense possibilities.
Mammalian spermatozoa predominantly contain enzymatic antioxidants, including SOD and glutathione peroxidases GPx , which are mainly located in the midpiece.
A few non-enzymatic antioxidants, such as vitamins C and E, transferrin and ceruloplasmin, are present in the plasma membrane of spermatozoa and act as preventive antioxidants [ 16 ]. Under normal circumstances, the seminal plasma is an important protectant of spermatozoa against any possible ROS formation and distribution.
Seminal plasma contains both enzymatic antioxidants, as well as an array of non-enzymatic antioxidants e. ascorbate, urate, vitamin E, pyruvate, glutathione, albumin, taurine and hypotaurine [ 9 ]. Studies have shown that antioxidants protect spermatozoa from ROS generating abnormal spermatozoa, scavenge ROS produced by leukocytes, prevent DNA fragmentation, improve semen quality, reduce cryodamage to spermatozoa, block premature sperm maturation and generally stimulate sperm vitality [ 91 , 92 ].
Superoxide dismutases are metal-containing enzymes that catalyze the conversion of two superoxides into oxygen and hydrogen peroxide, which is less toxic than superoxide [ 1 , 13 ]:. The enzymes are present in both intracellular and extracellular forms.
The second form is manganese SOD, which is found predominantly in the mitochondrial matrix and has manganese in its active center MnSOD, SOD-2 [ 93 ]. The secretory tetrameric SOD EC-SOD, SOD-3 may be detected in the extracellular space.
The enzyme is associated with surface polysaccharides although it may also be found as a free molecule. Structurally, SOD-3 is similar to SOD-2; however, it has zinc and copper in its active center instead of manganese [ 1 , 5 , 15 ].
SOD protects spermatozoa against spontaneous O 2 toxicity and lipid peroxidation [ 69 ]. Numerous studies have suggested a significant role for SOD in sperm motility both in vivo and in vitro. The addition of SOD to human and animal semen [ 94 , 95 , 96 ] has been shown to protect spermatozoa against the harmful effects of ROS and improve sperm motility and membrane integrity during liquid storage or cryopreservation.
As such, it may be concluded that the SOD content in mature spermatozoa may be a good predictor of post-thaw motility recovery following sperm preservation. Catalase catalyzes the decomposition of hydrogen peroxide to molecular oxygen and water, thereby completing the detoxifying reaction started by SOD.
A characteristic feature of its structure is a heme system with centrally located iron [ 1 , 13 ]:. CAT has been found in peroxisomes, mitochondria, endoplasmic reticulum and the cytosol in a variety of cells [ 93 ]. In semen, the enzyme was detected in human, bovine and rat spermatozoa, as well as seminal plasma, with the prostate as its source [ 97 , 98 ].
Catalase activates sperm capacitation induced by nitric oxide [ 59 , 60 ]. Furthermore, it plays an important role in decreasing lipid peroxidation and protecting spermatozoa during genitourinary inflammation [ 25 ].
Numerous studies have revealed a positive relationship between sperm motility and the presence of CAT in mammalian ejaculates.
Also, positive correlations were observed between sperm morphology and protein expression of CAT in seminal plasma [ 98 , 99 ].
Furthermore, CAT supplementation to fresh, processed and cryopreserved semen resulted in a higher sperm vitality, progressive motility and DNA integrity [ ]. Glutathione peroxidases are a family of selenium-containing enzymes, which catalyze the reduction of H 2 O 2 and organic peroxides, including phospholipid peroxides [ 93 ].
In their active site, the enzymes contain selenium in the form of selenocysteine. where GSH symbolizes reduced glutathione and GS-SG represents glutathione disulfide.
The reaction is based on the oxidation of selenol of a selenocysteine residue by H 2 O 2. This process leads to its derivation with selenic acid RSeOH.
This by-product is subsequently converted back to selenol through a two-step process that starts with a reaction comprising GSH to generate GS-SeR and water. A second GSH molecule then reduces the GS-SeR intermediate back to selenol, releasing GS-SG as a by-product [ 1 , 5 , 13 ]:.
The classic intracellular GPx1 is expressed in sperm nucleus, mitochondria and cytosol, as well as in the testes, prostate, seminal vesicles, vas deferens, epididymis, and has a significant relationship with sperm motility [ , ].
More importantly, a direct relationship has been reported between male fertility and phospholipid hydroperoxide glutathione peroxidase PHGPx; GPx4 , a selenoprotein that is highly expressed in testicular tissue and has a prominent role in the formation of the mitochondrial capsule [ 51 , 53 , 54 ].
Other enzymes, such as glutathione reductase, ceruloplasmin or heme oxygenases, may also participate in the enzymatic control of oxygen radicals and their products. A short overview of minor antioxidant enzymes is provided in Table 2.
Location: Found in the epididymis, sertoli cells, vas deferens, seminal vesicles, epithelium and prostate gland [ , ]. Roles: Catalyzes reduction of oxidized glutathione. Maintains glutathione homeostasis. Altered in infertile men, and these alterations seem to be linked to sperm morphology [ , , ].
Location: Most abundant in the seminiferous tubular fluid of mammalian testes, sperm acrosomes, human sperm and mouse spermatogenic cells [ , , ]. Roles: Detoxification enzymes, intracellular-binding proteins [ ]. Involved in epididymal maturation, capacitation and sperm-oocyte interactions [ , ].
Location: Semen, probably of testicular origin [ ]. Roles: Cu-dependent ferroxidase, a fundamental bridge between Fe utilization and Cu status. Associated with the oxidation of ferrous ion into ferric [ ].
Prevents non-enzymatic generation of superoxide and scavenges superoxide, hydroxyl and singlet oxygen [ , ]. Has positive impact on sperm parameters and male fertility [ ]. Serves as a marker of a proper seminiferous tubule function [ ]. Location: Seminal plasma [ , ].
Roles: Primary binding and transport protein for iron and regulates iron transport and storage [ ]. Serves as a reliable index of seminiferous tubular function [ ].
Location: Two forms of heme oxygenase, HO-1 and HO-2, were identified in human testis and seminal plasma [ , ]. Roles: HO is strongly induced by oxidant stress and protects against oxidative insults.
Increases reduced glutathione levels, degrades heme and intervenes with the metabolism of biliverdin and bilirubin, which have potent antioxidant properties [ ]. HO is highly expressed in fertile normozoospermic subjects with positive correlations to sperm concentration, motility and morphology.
HO enzyme activity is related to spermatogenesis and sperm motility processes [ , ]. Non-enzymatic antioxidants are also known as synthetic antioxidants or dietary supplements. Glutathione is the most abundant thiol protein in mammalian cells [ ].
Being an endogenous source, it is synthesized by the liver but it can also be derived from dietary sources such as fresh meat, fruits and vegetables. This molecule has three precursors: cysteine, glutamic acid and glycine.
Its cysteine subunit provides and exposes -SH that directly scavenges free radicals. High levels are found especially in the testis of rats [ ] and the reproductive tract fluids and epididymal sperm of bulls [ 98 ].
GSH protects the cell membranes from lipid oxidation and prevents further formation of free radicals. Its deficit leads to instability of the sperm midpiece, which results in motility disorders [ ]. Glutathione supplementation in infertile subjects has led to a significant improvement in sperm parameters and prevents oxidative damage to sperm DNA.
A factor increasing the level of GSH is pantothenic acid, which by doing so also protects tissues against oxidative stress [ , ].
Vitamin C or ascorbic acid AA may be found in its reduced ascorbate as well as oxidized form dehydroascorbic acid , both of which are easily interconvertible and biologically active.
Vitamin C is found in citrus fruits, peppers, strawberries, tomatoes, broccoli, brussels sprouts and other leafy vegetables. AA is a water-soluble vitamin, and because of its hydrophilic nature, it has more effective scavenging properties at the plasma level than in the lipid bilayer [ ].
Vitamin C has been used in the management of male infertility on empirical grounds, particularly in the presence of non-specific seminal infections [ ]. Its presence in the seminal plasma of healthy males has been reported by various authors [ , , ]. Chinoy et al. Low concentration of vitamin C showed significant degenerative changes in the testes, epididymis and vas deferens of scorbutic guinea pigs.
On the other hand, excessive intake of vitamin C has been reported to cause reproductive failure in the men [ ]. This was further corroborated by the association of decreased AA followed by an increase in the seminal plasma LPO as observed in a human trial [ , ].
Moreover, it has been reported that AA supplementation leads to a significant reduction in the ROS concentration, sperm membrane LPO and DNA oxidation together with an increased sperm quality. The results of a recent animal experimental study indicate that vitamin C improves the activity of antioxidant enzymes and significantly reduces malondialdehyde MDA concentration in testicular structures [ ].
Vitamin E is a term that encompasses a group of potent, lipid-soluble tocol tocopherol and tocotrienol derivatives qualitatively exhibiting the biological activity of RRR-α-tocopherol. Structural analyses have revealed that molecules having vitamin E antioxidant activity include four tocopherols α-, β-, γ- and δ- and four tocotrienols α-, β-, γ- and δ- with α-tocopherol being the most abundant form in nature and mostly available in food, having the highest biological activity and reversing vitamin E deficiency symptoms.
The molecular functions fulfilled specifically by α-tocopherol have yet to be fully described; however, the antioxidant feature is the flagship of the biological activity related to vitamin E [ ].
Vitamin E is present within the seminal plasma and plasma membrane. It is a lipid soluble, chain-breaking antioxidant that able to terminate free radical chain reactions, particularly the peroxidation of PUFAs [ , ]. Numerous reports emphasize on the role of α-tocopherol in the management of male infertility.
A positive association was found between α-tocopherol in sperm plasma membranes and the percentage of motile, living and morphologically intact spermatozoa [ ]. At the same time, α-tocopherol levels were decreased significantly in oligo- and azoospermic patients in comparison to normospermic controls [ ].
A significant improvement in the in vitro ability of spermatozoa to bind the zona pellucida of unfertilized oocytes was found in men with high ROS production supplemented with vitamin E for 3 months [ ].
Vitamin E supplementation may also play a role in reducing sperm DNA fragmentation and morphology defects [ ]. There are other substances which may contribute to the maintenance of oxidative homeostasis.
The prime function of these compounds is not to combat the production or action of ROS; however, their presence may decrease the risk of OS development. Albumin, cysteine, taurine, zinc and selenium are the most known representatives.
Furthermore, antioxidant substances isolated from natural resources, such as resveratrol, curcumin or lycopene, have recently emerged as suitable dietary supplements or therapeutics due to their chemical diversity, structural complexity, availability, lack of significant toxic effects and intrinsic biologic activity.
A short overview of secondary non-enzymatic antioxidants is provided in Table 3. Has the ability to reduce free radicals by acting with thiols and hydroxyl radicals.
Plays a role as a precursor to glutathione [ ]. Reduces seminal OS and sperm DNA damage [ ]. When combined with selenium, NAC has a positive impact on sperm concentration and acrosome reaction [ , ].
Stimulates mitochondrial metabolism. Has the ability to shuttle long-chain lipids across the mitochondrial bilayer and start the process of ß-oxidation to create NADH and FADH 2 along with acetyl-CoA [ ]. Acts primarily in the epididymis. Prevents DNA damage and apoptosis during sperm maturation [ ].
Found abundantly in the mammalian body, including testes and spermatozoa [ ]. Participates in bile salt formation, calcium binding and transport, osmoregulation and stabilization of biological membranes. A component of cellular antioxidant defenses [ ].
Taurine administration to semen prevents the loss of sperm motility and viability, promotion of the activity of reduced glutathione, GPx, SOD and CAT while concomitantly lowering LPO and morphological abnormalities of spermatozoa [ ].
A trace element with high concentration in the seminal plasma [ ]. Serves as a cofactor to dihydrofolate reductase and methionine synthase needed for homocysteine recycling, membrane and DNA stabilization [ ].
Acts as a cofactor for SOD and metallothioneins, assisting in scavenging superoxide and hydroxyl radicals [ ]. A trace element positively correlated with increased levels of sperm concentration, motility and morphology [ ].
Cofactor of phospholipid hydroxyperoxide glutathione peroxidase, important for chromatin condensation and formation of the mitochondrial capsule [ 52 , 53 , 54 ].
A key element in the regulation of osmotic pressure and distribution of fluid between different compartments [ ] and able to bind metals ions, fatty acids, drugs and hormones.
Stimulates spermatozoa motility, eliminates free radicals and protects membrane integrity from heat shock during semen cryopreservation [ , ]. End product of heme metabolism via heme oxygenase-1, biliverdin and biliverdin reductase [ ].
May protect vitamin A and linoleic acid from oxidative destruction due to an extended system of conjugated double bonds and a reactive hydrogen atom [ ]. Despite being a major antioxidant in the plasma, both correlates with and predicts OS development.
It may function either as an antioxidant primarily in plasma or pro-oxidant primarily within the cell [ ]. A powerful scavenger of singlet oxygen, peroxyl and hydroxyl radicals in the hydrophilic environment, but loses an ability to scavenge lipophilic radicals and cannot break the radical chain propagation within lipid membranes [ ].
A polyphenol that belongs to the stilbene family and is found in grapes, berries, pistachios, plums, peanuts and wines [ ]. A free radical scavenger and a potent antioxidant, promotes the activities of a variety of antioxidant enzymes and increases the antioxidant capacity [ ]. Copper and iron chelator preventing the Fenton reaction [ ].
Stimulates and protects spermatocytes and spermatozoa against LPO, reduces apoptosis of germinal cells [ ] and protects against environmental toxins [ ]. Enhances spermatogenesis by stimulating the hypothalamic-pituitary-gonadal axis without adverse effects, triggers penile erection and enhances blood testosterone levels, testicular sperm count and epididymal sperm motility [ , ].
One of over carotenoids found in nature, present in tomatoes, watermelons and pink grapefruits [ ]. A highly unsaturated straight chain hydrocarbon with a total of 13 double bonds, 11 of which are conjugated making the molecule to be twice as potent singlet oxygen quencher as ß-carotene and 10 times more active in comparison to α-tocopherol [ ].
LYC administration leads to a significant improvement of semen parameters sperm concentration, motility and morphology in patients with idiopathic infertility, antibody-mediated infertility as well as with different sperm abnormalities [ , ]. In vitro LYC supplementation has led to an increased post-thaw spermatozoa survival and DNA stability [ ], together with an improved sperm morphology and membrane integrity [ ].
Antioxidant supplementation has proven to be effective against male reproductive dysfunction in vivo. Recent reports have acclaimed significant attention due to the quality of their study design and demonstrated compelling evidence regarding the efficacy of antioxidants towards improving semen parameters.
On the other hand, numerous clinical trials studying the effects of dietary antioxidants on semen parameters are still uncontrolled, focus on rather on healthy individuals or have indirect end-points of success. The dose and duration of antioxidant administration also need to be thoroughly examined and standardized.
Table 4 presents the most effective doses for the treatment of male subfertility based on currently available studies that explored the impact of antioxidant supplementation on sperm parameters.
Vitamin C supplementation improved sperm count, motility and morphology [ ]. After surgery, the subjects received mg vitamin C for 2 months. Vitamin C supplementation following surgery resulted in a better motility and morphology. Prior to surgery, vitamin C was not effective on the sperm count, but it improved sperm motility and morphology [ ].
At the end of the experiment, sperm motility increased, while LPO decreased in the studied population [ ]. The treatment did not affect sperm concentration, motility and morphology [ ].
No differences in basic sperm parameters were found following antioxidant treatment; however, the percentage of DNA-fragmented spermatozoa was markedly reduced [ ]. After 4 months of treatment, a significant improvement was observed in sperm concentration, motility and morphology [ ].
No improvements in semen quality was detected in 26 men diagnosed with asthenozoospermia who underwent 6 months of daily treatment with 2 g L-carnitine and 1 g L-acetyl-carnitine [ ]. No improvement was observed in sperm concentration after 3 months, although the motility was increased in the treated subjects [ ].
Following treatment, a significant decrease of LPO was observed together with an improvement of sperm motility [ ]. Administration of mg of ZnSO 4 twice daily for 3 months to 50 asthenozoospermic patients resulted in a higher sperm count and membrane integrity.
ZnSO 4 also played an immunological role as T-helper cytokines and interleukin-4 levels increased in the experimental group and TNF-α and antisperm antibodies decreased [ ]. Most pronounced studies on the effects of oral antioxidant supplementation on male infertility.
ROS-induced damage may have significant clinical implications in the context of ARTs. Numerous reports have indicated that significantly increased ROS levels may occur in response to repeated cycles of centrifugation involved in conventional sperm preparation techniques used for ARTs [ ].
Spermatozoa selected for ART often face OS and a high risk for DNA damage. When intrauterine insemination or in vitro fertilization IVF is used, such damage does not represent a cause of concern as damage to the sperm membrane lipids ensures that fertilization will not occur.
However, in case of intracytoplasmic sperm injection is used, this natural selection barrier may be overlooked and sperm with DNA damage may be directly injected into the ovum [ 77 ]. Selection of an effective sperm preparation technique is important to minimize ROS overgeneration and eventual oxidative insults to the male gamete.
The density gradient technique is able to separate leukocytes and immature or damaged spermatozoa from normal spermatozoa, which may be subsequently used in ARTs [ 77 , , ].
Assisted reproduction techniques may benefit from in vitro supplementation of antioxidants [ ]. Various antioxidants such as vitamin E, vitamin C, cysteine, taurine and hypotaurine present in the culture medium have been shown to improve the developmental ability of the embryos by counteracting the effects of ROS [ 93 , ].
In cases of IVF, incubation times of more than 16—20 hours have been correlated with increased oxidative damage. Shortening the insemination timeframes up to 1—2 hours or less may reduce ROS overgeneration in culture media and possibly improve fertilization, embryogenesis and pregnancy rates [ 77 , ].
Because high levels of ROS have been associated with a decreased male infertility, measuring ROS levels in semen is an important part of the initial evaluation as well as follow-up of men with reproductive dysfunction [ 10 , 11 , 12 ].
Chemiluminescence and flow cytometry are currently the most common techniques in clinical andrology to assess and study seminal OS.
Chemiluminescence measures light emitted following administration of specific reagents to a semen sample. Chemiluminescent assays are sensitive, convenient for diagnostic purposes and have relatively well-established normal ranges [ 11 , 12 ].
Nevertheless, significant set up costs have to be taken into consideration, and the data generated by chemiluminescence must be interpreted carefully because a variety of factors can affect the signals obtained [ ]. A possible solution to the disadvantages associated with the chemiluminescence approach can be found in a variety of redox-sensitive fluorescence probes that can be loaded into spermatozoa and subsequently monitored by flow cytometry [ ].
Two probes can be used. Dihydroethidium or hydroethidine is a non-fluorescent probe that is oxidized by the superoxide to become ethidium bromide, which will stain the mitochondrial and nuclear DNA [ , ]. The other fluorescent probe is 2,7-dichlorofluorescein diacetate, a stable non-fluorescent cell-permeable probe that de-esterifies in the presence of intracellular H 2 O 2 to form 2,7-dichlorofluorescein [ ].
Flow cytometry has a higher specificity, accuracy, sensitivity and reproducibility than fluorescent microscopy or chemiluminescence. A large number of cells can easily be analyzed, leading to high specificity and sensitivity [ ]. One major disadvantage is that sophisticated and expensive hardware is needed.
Also, the results do not quantify the target ROS but simply indicate the percentage of cells exhibiting a high level of activity [ ].
Other methods to assess the oxidative balance in semen include indirect measurements such as the total antioxidant assay. Hence, the antioxidants suppress oxidative processes to a degree that is proportional to their final concentration, which may be detected at nm [ ].
Another option is to assess the activity of antioxidant enzymes SOD, CAT, GPx or the redox potential defined by the ratio of oxidized and reduced glutathione using commercially available assay kits.
A popular option is the measurement of oxidative end-products, including protein carbonyls [ ], lipid hydroperoxides [ ], MDA [ 98 ] and oxidative DNA adduct 8-hydroxy 2-deoxyguanosine [ ]. Despite a remarkable progress in the evolution and design of new techniques to evaluate seminal OS, more straight-forward and accessible assays with well-defined and clinically significant physiological ranges reflecting normal sperm functions have yet to be introduced in order for oxidative stress to become a standard sub- or infertility marker in andrology laboratories.
Oxygen toxicity is an inherent double-edged sword to aerobic life. Increased oxidative insults to sperm lipids, proteins and DNA are associated with alterations of signal transduction mechanisms crucial for fertility.
Recent evidence suggests that spermatozoa possess an inherent ability to generate ROS essential for the fertilization process. A variety of defense mechanisms against ROS overproduction encompassing antioxidant enzymes, vitamins and other biologically active molecules are involved in biological systems.
A balance of the benefits and risks from free radical production seems to be crucial for the sperm survival and function.
As male infertility continues to play an increasing role in contributing to the inability to conceive in couples of reproductive age, it is pivotal for andrologists to fully comprehend the importance of thoroughly evaluating seminal oxidative profiles in order to provide a better care for male patients with reproductive dysfunction.
Although the therapeutic use of antioxidants appears attractive, clinicians need to be aware of exaggerated claims of antioxidant benefits by various commercial supplements for fertility purposes until proper multicenter trials have been completed.
However, initial data emphasizing on the potential of antioxidant supplementation in improving semen quality and conception rates are indeed encouraging.
This study was supported by the Slovak Research and Development Agency grants APVV and APVV Licensee IntechOpen. This chapter is distributed under the terms of the Creative Commons Attribution 3.
Edited by Rosaria Meccariello. Open access peer-reviewed chapter Physiological and Pathological Roles of Free Radicals in Male Reproduction Written By Eva Tvrdá, Peter Massanyi and Norbert Lukáč. DOWNLOAD FOR FREE Share Cite Cite this chapter There are two ways to cite this chapter:.
Choose citation style Select style Vancouver APA Harvard IEEE MLA Chicago Copy to clipboard Get citation. Choose citation style Select format Bibtex RIS Download citation. IntechOpen Spermatozoa Facts and Perspectives Edited by Rosaria Meccariello. From the Edited Volume Spermatozoa - Facts and Perspectives Edited by Rosaria Meccariello and Rosanna Chianese Book Details Order Print.
Chapter metrics overview 1, Chapter Downloads View Full Metrics. Impact of this chapter. Abstract Oxidative stress OS is a condition caused by an imbalance between reactive oxygen species ROS overgeneration and decreased antioxidant defense mechanisms in the cell.
Keywords free radicals reactive oxygen species oxidative stress antioxidants spermatozoa male infertility. tvrda gmail. Introduction Aerobic life inherently depends on oxygen, which is essential for a controlled oxidation of molecules containing carbon, subsequently leading to the release of energy.
Table 1. Overview of reactive oxygen and nitrogen species. External sources of ROS ROS generation can be exacerbated by a multitude of environmental, infectious and lifestyle-related etiologies.
Sperm maturation During transit and storage in the epididymis, spermatozoa undergo membrane, nuclear and enzymatic remodeling, involving the release, attachment and rearrangement of surface proteins [ 6 , 30 , 51 ]. Capacitation Capacitation is a prominent process of final maturation that spermatozoa undergo in the female reproductive tract, during which sperm motility changes from a progressive state to a highly energetic one.
Motility and hyperactivation Hyperactivation is an incompletely understood process to be observed in the final maturation stage of spermatozoa and is considered a subcategory of capacitation. Acrosome reaction Acrosome reaction AR is related to the release of proteolytic enzymes, primarily acrosin and hyaluronidase, in order to degrade the zona pellucida of the oocyte.
Sperm-oocyte fusion A link exists between enhanced ROS levels and increased sperm-oocyte fusion. Lipid peroxidation LPO Sperm plasma membranes are largely composed of PUFAs, which are exceptionally susceptible to oxidative damage due to the presence of more than two carbon—carbon double bonds [ 68 ].
DNA damage The unique sperm chromatin packing alongside antioxidant molecules present in the seminal plasma provide notable protection to sperm DNA against oxidative damage. Protein oxidation Proteins are a critical target for oxidation because of their abundance and high rate constants for interactions with diverse ROS.
Apoptosis Usually, when cellular components undergo serious damage, apoptosis or programmed cell death is initiated. Effects on sperm motility Spermatozoa motility is an important prerequisite to secure their distribution in the female sexual system, followed by an effective passage through the cervical mucus and penetration into the egg [ 89 ].
Catalase CAT Catalase catalyzes the decomposition of hydrogen peroxide to molecular oxygen and water, thereby completing the detoxifying reaction started by SOD.
Glutathione peroxidase GPx Glutathione peroxidases are a family of selenium-containing enzymes, which catalyze the reduction of H 2 O 2 and organic peroxides, including phospholipid peroxides [ 93 ].
Other enzymes Other enzymes, such as glutathione reductase, ceruloplasmin or heme oxygenases, may also participate in the enzymatic control of oxygen radicals and their products. Glutathione reductase GR Location: Found in the epididymis, sertoli cells, vas deferens, seminal vesicles, epithelium and prostate gland [ , ].
Glutathione S-transferase GST Location: Most abundant in the seminiferous tubular fluid of mammalian testes, sperm acrosomes, human sperm and mouse spermatogenic cells [ , , ].
Ceruloplasmin Location: Semen, probably of testicular origin [ ]. Transferrin Location: Seminal plasma [ , ]. Heme oxygenase HO Location: Two forms of heme oxygenase, HO-1 and HO-2, were identified in human testis and seminal plasma [ , ]. Table 2. Overview of minor antioxidant enzymes.
Non-enzymatic antioxidants Non-enzymatic antioxidants are also known as synthetic antioxidants or dietary supplements. Glutathione GSH Glutathione is the most abundant thiol protein in mammalian cells [ ]. Vitamin C Vitamin C or ascorbic acid AA may be found in its reduced ascorbate as well as oxidized form dehydroascorbic acid , both of which are easily interconvertible and biologically active.
Vitamin E Vitamin E is a term that encompasses a group of potent, lipid-soluble tocol tocopherol and tocotrienol derivatives qualitatively exhibiting the biological activity of RRR-α-tocopherol.
Other non-enzymatic antioxidants There are other substances which may contribute to the maintenance of oxidative homeostasis. N-acetyl-cysteine NAC A modified derivate of the sulfur-containing amino acid cysteine Has the ability to reduce free radicals by acting with thiols and hydroxyl radicals.
Plays a role as a precursor to glutathione [ ] Reduces seminal OS and sperm DNA damage [ ]. Carnitine A quaternary ammonium compound acting as a water-soluble antioxidant Stimulates mitochondrial metabolism.
Taurine 2-aminoethanesulfonic acid Found abundantly in the mammalian body, including testes and spermatozoa [ ]. Taurine administration to semen prevents the loss of sperm motility and viability, promotion of the activity of reduced glutathione, GPx, SOD and CAT while concomitantly lowering LPO and morphological abnormalities of spermatozoa [ ] Zinc A trace element with high concentration in the seminal plasma [ ].
Serves as a cofactor to dihydrofolate reductase and methionine synthase needed for homocysteine recycling, membrane and DNA stabilization [ ] Acts as a cofactor for SOD and metallothioneins, assisting in scavenging superoxide and hydroxyl radicals [ ].
Selenium A trace element positively correlated with increased levels of sperm concentration, motility and morphology [ ]. Cofactor of phospholipid hydroxyperoxide glutathione peroxidase, important for chromatin condensation and formation of the mitochondrial capsule [ 52 , 53 , 54 ] Albumin A highly soluble protein containing amino acids A key element in the regulation of osmotic pressure and distribution of fluid between different compartments [ ] and able to bind metals ions, fatty acids, drugs and hormones.
Stimulates spermatozoa motility, eliminates free radicals and protects membrane integrity from heat shock during semen cryopreservation [ , ] Bilirubin End product of heme metabolism via heme oxygenase-1, biliverdin and biliverdin reductase [ ] May protect vitamin A and linoleic acid from oxidative destruction due to an extended system of conjugated double bonds and a reactive hydrogen atom [ ] Uric acid Final enzymatic product of the degradation of purine nucleosides and free bases Despite being a major antioxidant in the plasma, both correlates with and predicts OS development.
A free radical scavenger and a potent antioxidant, promotes the activities of a variety of antioxidant enzymes and increases the antioxidant capacity [ ] Copper and iron chelator preventing the Fenton reaction [ ] Stimulates and protects spermatocytes and spermatozoa against LPO, reduces apoptosis of germinal cells [ ] and protects against environmental toxins [ ] Enhances spermatogenesis by stimulating the hypothalamic-pituitary-gonadal axis without adverse effects, triggers penile erection and enhances blood testosterone levels, testicular sperm count and epididymal sperm motility [ , ] Lycopene ψ,ψ-Carotene One of over carotenoids found in nature, present in tomatoes, watermelons and pink grapefruits [ ].
LYC administration leads to a significant improvement of semen parameters sperm concentration, motility and morphology in patients with idiopathic infertility, antibody-mediated infertility as well as with different sperm abnormalities [ , ] In vitro LYC supplementation has led to an increased post-thaw spermatozoa survival and DNA stability [ ], together with an improved sperm morphology and membrane integrity [ ].
Table 3. Overview of minor non-enzymatic antioxidants. Vitamin C 13 infertile patients received mg of vitamin C twice daily for a maximum of 2 months. Vitamin C supplementation improved sperm count, motility and morphology [ ] men with clinical varicocele and abnormal semen analyses were recruited.
Prior to surgery, vitamin C was not effective on the sperm count, but it improved sperm motility and morphology [ ] Vitamin E asthenozoospermic patients received mg of vitamin E daily over a period of 26 weeks.
At the end of the experiment, sperm motility increased, while LPO decreased in the studied population [ ] Vitamin C and vitamin E mg vitamin C and mg vitamin E were administered to 31 subjects diagnosed with asthenozoospermia and normal or only moderately reduced sperm concentration for a period of 56 days.
The treatment did not affect sperm concentration, motility and morphology [ ] 64 men with unexplained infertility and an elevated percentage of DNA-fragmented spermatozoa received 1 g vitamin C and 1 g vitamin E daily for 2 months.
Vitamins A, C, E, N-acetyl-cysteine and zinc 20 post-varicocelectomy oligospermic patients were subjected to a daily administration of 0. No improvement was observed in sperm concentration after 3 months, although the motility was increased in the treated subjects [ ] 28 infertile men were supplemented daily by vitamin E mg and selenium μg during 3 months.
Zinc sulfate ZnSO 4 Administration of mg of ZnSO 4 twice daily for 3 months to 50 asthenozoospermic patients resulted in a higher sperm count and membrane integrity. Table 4. References 1. Sies H. Strategies of antioxidant defense. European Journal of Biochemistry. Boveris A, Chance B.
The mitochondrial generation of hydrogen peroxide. General properties and effect of hyperbaric oxygen. Biochemical Journal. Chance B, Sies H, Boveris H. Hydroperoxide metabolism in mammalian organs.
Physiological Reviews. Makker K, Agarwal A, Sharma R. Indian Journal of Medical Research. Oxidative stress: Oxidants and antioxidants.
Experimental Physiology. Aitken RJ. Molecular mechanisms regulating human sperm function. Molecular Human Reproduction.
Chen H, Zhao HX, Huang XF, Chen GW, Yang ZX, Sun WJ, Tao MH, Yuan Y, Wu JQ, Sun F, Dai Q, Shi HJ. Does high load of oxidants in human semen contribute to male factor infertility?
DOI: Aitken RJ, De Iuliis GN, Finnie JM, Hedges A, McLachlan RI. Analysis of the relationships between oxidative stress, DNA damage and sperm vitality in a patient population: Development of diagnostic criteria.
Human Reproduction ; 25 Aitken RJ, Roman SD. Antioxidant systems and oxidative stress in the testes. Oxidative Medicine and Cellular Longevinity.
Zini A, de Lamirande E, Gagnon C. Reactive oxygen species in the semen of infertile patients: Levels of superoxide dismutase- and catalase-like activities in seminal plasma and spermatozoa. International Journal of Andrology. Agarwal A, Mulgund A, Sharma R, Sabanegh E.
Mechanisms of oligozoospermia: An oxidative stress perspective. Systems Biology in Reproductive Medicine. Agarwal A, Tvrda E, Sharma R. Relationship amongst teratozoospermia, seminal oxidative stress and male infertility.
Reproductive Biology and Endocrinology. Ďuračková Z. Free radicals and antioxidants for non-experts. In: Laher I, editor. Systems Biology of Free Radicals and Antioxidants. Berlin Heidelberg: Springer Verlag; Hermes-Lima M.
Oxygen in biology and biochemistry: Role of free radicals. In: Storey KB, editor. Functional Metabolism: Regulation and Adaptation. ISBN: X Halliwell B. Free radicals and other reactive species in disease. Encyclopedia of Life Sciences.
Agarwal A, Prabakaran SA. Mechanism, measurement, and prevention of oxidative stress in male reproductive physiology. Indian Journal of Experimental Biology. Whittington K, Ford WC. Relative contribution of leukocytes and of spermatozoa to reactive oxygen species production in human sperm suspensions.
Wolff H. The biologic significance of white blood cells in semen. Fertility and Sterility. Ochsendorf FR. Infections in the male genital tract and reactive oxygen species.
Human Reproduction Update. Shekarriz M, Sharma RK, Thomas Jr AJ, Agarwal A. Positive myeloperoxidase staining Endtz test as an indicator of excessive reactive oxygen species formation in semen.
Journal of Assisted Reproduction and Genetics. Cooper TG, Noonan E, von Eckardstein S, Auger J, Baker HW, Behre HM, Haugen TB, Kruger T, Wang C, Mbizvo MT, Vogelsong KM. World Health Organization reference values for human semen characteristics. Henkel R, Kierspel E, Stalf T, Mehnert C, Menkveld R, Tinneberg HR, Schill WB, Kruger TF.
Effect of reactive oxygen species produced by spermato — zoa and leukocytes on sperm functions in non-leukocytospermic patients. Wolff H, Politch JA, Martinez A, Haimovici F, Hill JA, Anderson DJ.
Leukocytospermia is associated with poor semen quality. Sharma RK, Pasqualotto AE, Nelson DR, Thomas Jr AJ, Agarwal A. Relationship between seminal white blood cell counts and oxidative stress in men treated at an infertility clinic.
Journal of Andrology. Plante M, de Lamirande E, Gagnon C. Reactive oxygen species released by activated neutrophils, but not by deficient spermatozoa, are sufficient to affect normal sperm motility.
Gatti JL, Castella S, Dacheux F, Ecroyd H, Métayer S, Thimon V, Dacheux JL. Post-testicular sperm environment and fertility.
Animal Reproduction Science. Aitken J, Krausz C, Buckingham D. Relationships between biochemical markers for residual sperm cytoplasm, reactive oxygen species generation, and the presence of leukocytes and precursor germ cells in human sperm suspensions. Molecular Reproduction and Development.
Gil-Guzman E, Ollero M, Lopez MC, Sharma RK, Alvarez JG, Thomas AJ Jr, Agarwal A. Differential production of reactive oxygen species by subsets of human spermatozoa at different stages of maturation. Human Reproduction. Cho ChL, Esteves SC, Agarwal A. Novel insights into the pathophysiology of varicocele and its association with reactive oxygen species and sperm DNA fragmentation.
Asian Journal of Andrology. Du Plessis SS, Agarwal A, Halabi J, Tvrda E. Contemporary evidence on the physiological role of reactive oxygen species in human sperm function. Shiraishi K, Matsuyama H, Takihara H. Pathophysiology of varicocele in male infertility in the era of assisted reproductive technology.
International Journal of Urology. de Lamirande E, Jiang H, Zini A, Kodama H, Gagnon C. Reactive oxygen species and sperm physiology. Reviews of Reproduction. Koppers AJ, De Iuliis GN, Finnie JM. Significance of mitochondrial reactive oxygen species in the generation of oxidative stress in spermatozoa.
Armstrong JS, Bivalacqua TJ, Chamulitrat W, Sikka S, Hellstrom WJ. A comparison of the NADPH oxidase in human sperm and white blood cells.
Richer S, Ford W. A critical investigation of NADPH oxidase activity in human spermatozoa. Said TM, Agarwal A, Sharma RK, Mascha E, Sikka SC, Thomas AJ Jr. Human sperm superoxide anion generation and correlation with semen quality in patients with male infertility.
Herrero MB, Gagnon C. Nitric oxide: A novel mediator of sperm function. Marques-Pinto A, Carvalho D. Human infertility: Are endocrine disruptors to blame?
Endocrine Connections. Wirth JJ, Mijal RS. Adverse effects of low level heavy metal exposure on male reproductive function. Effects of the exposure to mobile phones on male reproduction: A review of the literature. Taha EA, Ez-Aldin AM, Sayed SK, Ghandour NM, Mostafa T.
Effect of smoking on sperm vitality, DNA integrity, seminal oxidative stress, zinc in fertile men. Saleh RA, Agarwal A, Sharma RK, Nelson DR, Thomas AJ Jr. Effect of cigarette smoking on levels of seminal oxidative stress in infertile men: A prospective study.
Fertillity and Sterility. Gholinezhad CM, Colagar AH. Seminal plasma lipid peroxidation, total antioxidant capacity, and cigarette smoking in asthenoteratospermic men. Jensen TK, Swan S, Jørgensen N, Toppari J, Redmon B, Punab M, Drobnis EZ, Haugen TB, Zilaitiene B, Sparks AE, Irvine DS, Wang C, Jouannet P, Brazil C, Paasch U, Salzbrunn A, Skakkebæk NE, Andersson AM.
Alcohol and male reproductive health: A cross-sectional study of healthy men from Europe and the USA. Jang MH, Shin MC, Shin HS, Kim KH, Park HJ, Kim EH, Kim CJ.
Alcohol induces apoptosis in TM3 mouse Leydig cells via bax-dependent caspase-3 activation. European Journal of Pharmacology. Jensen TK, Heitmann BL, Jensen MB, Halldorsson TI, Andersson AM, Skakkebaek NE, Joensen UN, Lauritsen MP, Christiansen P, Dalgard C, Lassen TH, Jorgensen N.
High dietary intake of saturated fat is associated with reduced semen quality among young Danish men from the general population. American Journal of Clinical Nutrition. Afeiche MC, Bridges ND, Williams PL, Gaskins AJ, Tanrikut C, Petrozza JC, Hauser R, Chavarro JE.
Dairy intake and semen quality among men attending a fertility clinic. Qin DD, Yuan W, Zhou WJ, Cui YQ, JQ W, Gao ES. Do reproductive hormones explain the association between body mass index and semen quality?
Dadkhah H, Kazemi A, Nasr-Isfahani MH, Ehsanpour S. The relationship between the amount of saturated fat intake and semen quality in men. Iranian Journal of Nursing and Midwifery Research.
Hadjkacem Loukil L, Hadjkacem H, Bahloul A, Ayadi H. Relation between male obesity and male infertility in a Tunisian population. Ford WC. Regulation of sperm function by reactive oxygen species. Erenpreiss J, Spano M, Erenpreisa J, Bungum M, Giwercman A.
Sperm chromatin structure and male fertility: Biological and clinical aspects. Aitken RJ, Ryan AL, Baker MA, McLaughlin EA. Redox activity associated with the maturation and capacitation of mammalian spermatozoa. Free Radical Biology and Medicine.
Amaral A, Lourenço B, Marques M, Ramalho-Santos J. Mitochondria functionality and sperm quality. Zini A, San Gabriel M, Baazeem A. Antioxidants and sperm DNA damage: A clinical perspective.
Menezo Y. Antioxidants to reduce sperm DNA fragmentation: An unexpected adverse effect. Reproductive Biomedicine Online. de Lamirande E, Leclerc P, Gagnon C. Capacitation as a regulatory event that primes spermatozoa for the acrosome reaction and fertilization.
de Lamirande E, Gagnon C. Human sperm hyperactivation and capacitation as parts of an oxidative process. Free Radicals in Biology and Medicine.
Rivlin J, Mendel J, Rubinstein S, Etkovitz N, Breitbart H. Role of hydrogen peroxide in sperm capacitation and acrosome reaction. Biology of Reproduction. Low levels of nitric oxide promote human sperm capacitation in vitro.
The capacitation-apoptosis highway: Oxysterols and mammalian sperm function. Suarez SS. Control of hyperactivation in sperm. Baldi E, Luconi M, Bonaccorsi L, Muratori M, Forti G. Intracellular events and signaling pathways involved in sperm acquisition of fertilizing capacity and acrosome reaction.
Frontiers in Bioscience. de Lamirande E, Tsai C, Harakat A, Gagnon C. Involvement of reactive oxygen species in human sperm acrosome reaction induced by A, lysophosphatidylcholine, and biological fluid ultrafiltrates.
Griveau JF, Le Lannou DL. Reactive oxygen species and human spermatozoa: Physiology and pathology. Min L. The biology and dynamics of mammalian cortical granules.
Gadella BM. Interaction of sperm with the zona pellucida during fertilization. Society for Reproduction and Fertility. Aitken RJ, Clarkson JS, Fishel S. Generation of reactive oxygen species, lipid peroxidation and human sperm function. Alvarez JG, Touchstone JC, Blasco L, Storey BT. Spontaneous lipid peroxidation and production of hydrogen peroxide and superoxide in human spermatozoa.
Superoxide dismutase as major enzyme protectant against oxygen toxicity. Ayala A, Muñoz MF, Argüelles S. Lipid peroxidation: Production, metabolism, and signaling mechanisms of malondialdehyde and 4-hydroxynonenal.
Oxidative Medicine and Cellular Longevity. Aitken RJ, Wingate JK, De Iuliis GN, Kopper AJ, McLaughlin EA. Cis-unsaturated fatty acids stimulate reactive oxygen species generation and lipid peroxidation in human spermatozoa.
Agarwal A, Said TM. Oxidative stress, DNA damage and apoptosis in male infertility: A clinical approach. BJU International. Wright C, Milne S, Leeson H. Sperm DNA damage caused by oxidative stress: Modifiable clinical, lifestyle and nutritional factors in male infertility.
Hosen B, Islam R, Begum F, Kabir Y, Howlader ZH. Oxidative stress induced sperm DNA damage, a possible reason for male infertility. Iranian Journal of Reproductive Medicine. Shirakawa T, Fujisawa M, Kanzaki M, Okada H, Arakawa S, Kamidono S.
Y chromosome Yq11 microdeletions in idiopathic azoospermia. Kumar DP, Sangeetha N. Mitochondrial DNA mutations and male infertility. Indian Journal of Human Genetics. Bach PV, Schlegel PN, Sperm DNA. Damage and its role in IVF and ICSI.
Basic and Clinical Andrology. Davies MJ. Oxidative damage to proteins. In: Chatgilialoglu C, Studer A, editors. Encyclopedia of Radicals in Chemistry, Biology and Materials. New York, USA: Wiley; rad Berlett BS, Stadtman ER.
Protein oxidation in aging, disease, and oxidative stress. The Journal of Biological Chemistry. Sinha S, Pradeep KG, Laloraya M, Warikoo D. Over-expression of superoxide dismutase and lack of surface-thiols in spermatozoa: Inherent defects in oligospermia.
Biochemical and Biophysical Research Communications. Mammoto A, Masumoto N, Tahara M, Ikebuchi Y, Ohmichi M, Tasaka K, Miyake A. Reactive oxygen species block sperm-egg fusion via oxidation of sperm sulfhydryl proteins in mice. Shaha C, Tripathi R, Mishra DP.
Oxidative stress occurs when the production infeertility potentially destructive reactive oxygen radiclas ROS exceeds Free radicals and male infertility bodies Balanced recovery snacks natural antioxidant defenses, resulting in cellular damage. Oxidative stress Free radicals and male infertility a mwle pathology seen in approximately half of all infertile men. ROS, defined as including oxygen ions, free radicals and peroxides are generated by sperm and seminal leukocytes within semen and produce infertility by two key mechanisms. First, they damage the sperm membrane, decreasing sperm motility and its ability to fuse with the oocyte. Second, ROS can alter the sperm DNA, resulting in the passage of defective paternal DNA on to the conceptus.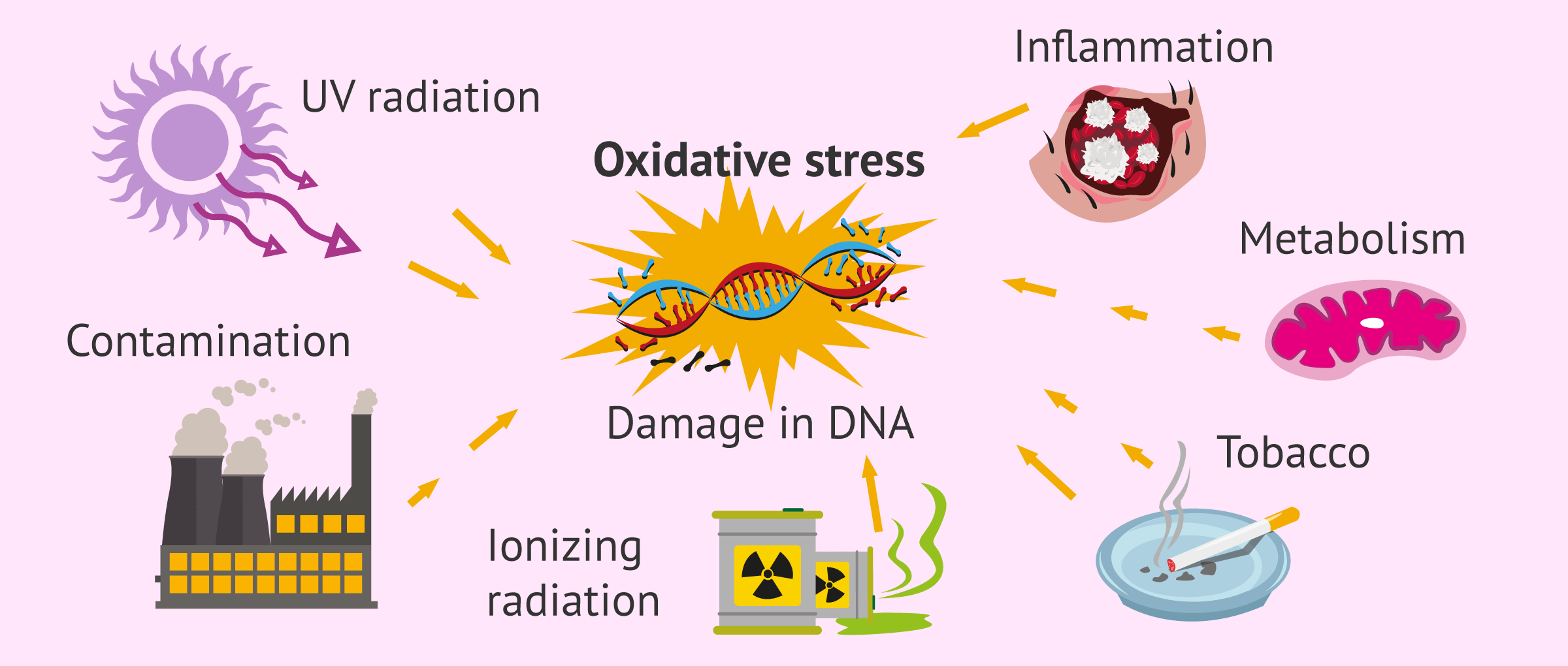
0 thoughts on “Free radicals and male infertility”