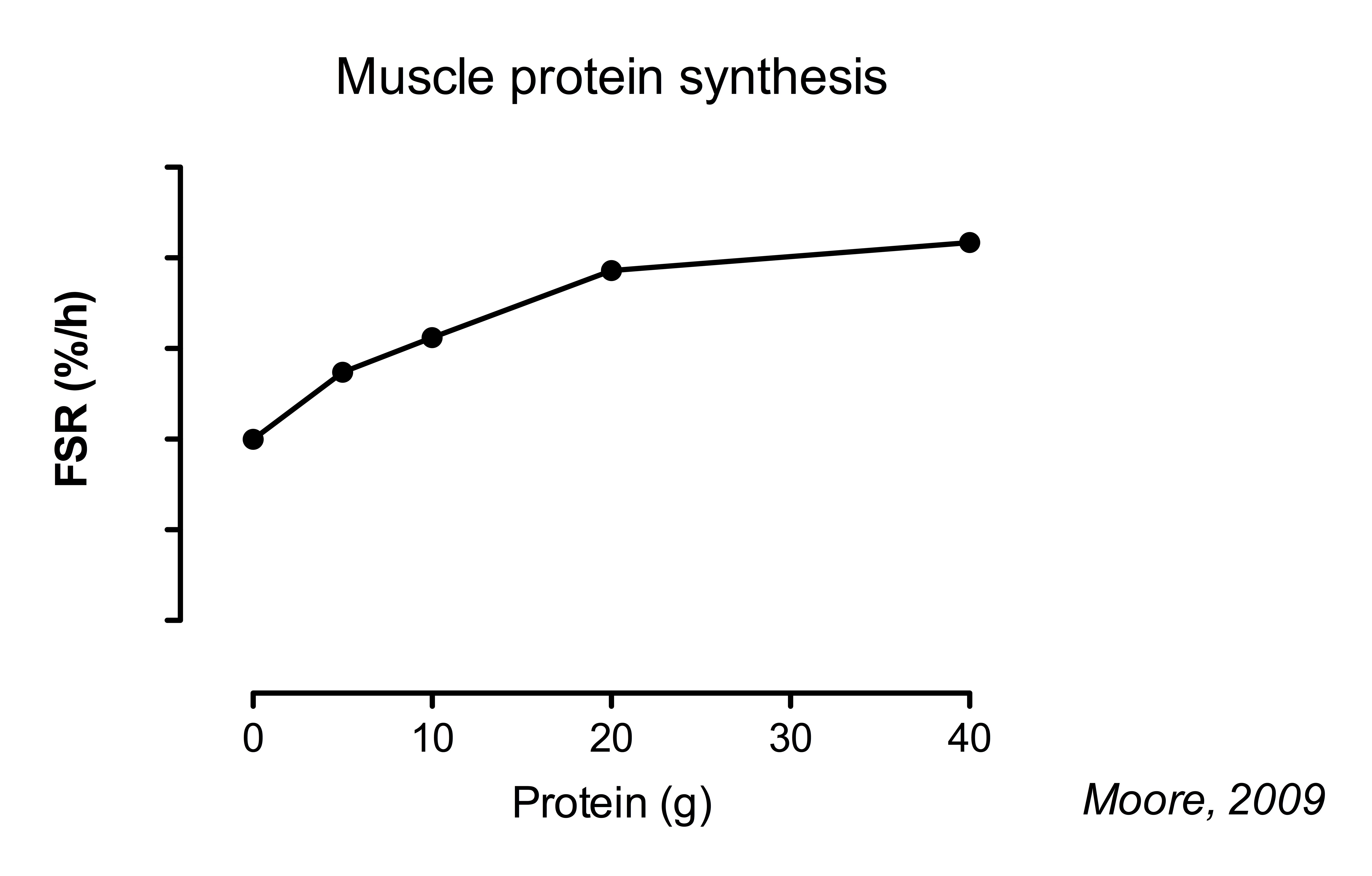
Protein and muscle protein synthesis in athletes -
In practice, this method utilizes stable isotope labeled amino acids i. Traditionally, FSR was calculated for mixed muscle proteins, that is, all muscle protein fractions combined. Methodological advances during the s allowed for the separation of muscle protein fractions Hasten et al.
Another recent advancement in the field is centered around the re-emergence of the orally administered deuterium oxide D 2 O tracer method to measure free-living integrative rates of MPS. Today, separation techniques have evolved further to measure FSR at the individual muscle protein level using D 2 O.
The focus of the review is on studies that directly determined MPS using the measurement of FSR. The controversy surrounding the value of acute i. More recently, an elegant study by Mitchell et al.
This study was novel in examining the within-participant i. No measurement of muscle protein breakdown was conducted in this study. As such, this study design offered insight into whether any heterogeneity in the muscle hypertrophic response to RET could be explained by differences in the acute response of MPS to REx between the 23 participants that conducted the study.
The muscle hypertrophic response was determined by measurement of pre—post RET changes in quadriceps volume and lean body mass using magnetic resonance imaging and dual-energy X-ray absorptiometry, respectively. The acute response of MPS was measured over a 6-hr recovery period following the first of 64 bout of REx.
Perhaps surprisingly to many at the time, and refuting their original hypothesis, the study by Mitchell et al. Moreover, no correlation of the change in MPS from rest with the change in muscle volume was reported Mitchell et al.
Indeed, this observation is consistent with the results of a comparable 16 weeks RET study by Mayhew et al. In this study, no relationship was observed between the acute response of mixed MPS measured in the fasted state 24 hr after the initial bout of REx and muscle hypertrophy as determined by measurement of muscle fiber cross-sectional area.
Taken together, these data suggest that acute measurements of MPS offer limited quantitative value for predicting individualized chronic changes in muscle mass following progressive RET, at least when the acute response of MPS is measured following the initial exercise session of the RET period.
These studies have contributed to some confusion—particularly for practitioners, students, and others without specialist knowledge of the strengths and limitation of stable isotope methodology—and controversy over the interpretation of data from the measurement of MPS in response to exercise and nutrition Mitchell et al.
In contrast, multiple lines of evidence support the notion that the acute response of MPS to REx, with or without nutritional intervention, is predictive of chronic changes in muscle mass with RET when repeatedly exposed to a comparable exercise or nutritional intervention, at least when studied on an averaged, group basis.
First, ingesting an 18 g bolus of milk protein immediately after REx stimulated a greater acute response of MPS than a dose-matched soy protein beverage in young men Wilkinson et al.
This finding was consistent with a longitudinal training study that reported a greater change in muscle hypertrophy when a milk protein beverage was consumed immediately after each REx session of a week RET program versus a soy protein beverage in young men Hartman et al.
Similarly, the greater acute response of MPS to ingesting 20 g of whey protein versus casein immediately post REx Tang et al.
Second, the acute response of MPS to REx when manipulating exercise workload low vs. high Burd, West, et al. Finally, REx-induced increases in putative anabolic hormones were not shown to increase the acute response of MPS West et al. When combined with data generated by Mitchell et al.
In our view, and that of others Damas et al. Several physiological factors, related to both the acute response of MPS to REx and the muscle hypertrophic response to RET, appear to contribute to the observed discrepancy between measured rates of MPS and muscle hypertrophy. Muscle hypertrophy is a complex physiological process that is altered as training progresses.
For the initial response of MPS to predict subsequent muscle hypertrophy during RET, it must be assumed that the measured response of MPS to REx is uniform throughout the training period.
However, it is clear that the response of MPS is modified from the initiation of RET and as training progresses Kim et al. This modification takes place on a number of levels that include the timecourse amplitude and duration and nature directed to anabolic or nonanabolic processes of the MPS response, as discussed below.
There is considerable evidence from both cross-sectional Phillips et al. In the untrained state, the acute response of MPS has been shown to peak later, but remain elevated for longer, after REx compared with the trained state Phillips et al.
Conversely, in the trained state, the acute response of MPS to REx is more rapid but shorter lived than the untrained state Phillips et al.
As a result, the overall acute stimulation of MPS after REx is generally considered to be greater in untrained versus trained individuals, at least when the absolute workload of REx is matched between training states Damas et al.
Given that training status clearly modulates the acute response of MPS to REx, it follows that the relationship between the acute MPS response to REx and chronic muscle growth response to RET may be altered over the time course of the training process.
To date, the most comprehensive study to examine the influence of training status on the relationship between the acute MPS response to REx and the muscle growth response to RET was conducted by Damas et al.
The RET program was divided into three phases, namely the initial i. Measurements of the acute MPS response to REx and muscle mass were obtained at each phase of RET. This elegant study design offered unique insight into the temporal relationship between acute measurements of MPS in response to REx, assessed in both the trained and untrained state, and the subsequent muscle growth response during RET.
The study by Damas, Phillips, Libardi, et al. In this regard, no relationship was observed between the acute response of myofibrillar—MPS to the initial REx bout of the RET period and the change in muscle mass following 10 weeks of RET.
As detailed above, this observation is consistent with previous studies that reported no association between the acute response of MPS to the initial REx bout and the change in muscle volume Mitchell et al.
In contrast, the acute response of MPS to REx measured at Weeks 3 and 10 were associated with chronic changes in muscle mass over the week RET period Damas, Phillips, Libardi, et al.
These data are consistent with recent studies that reported associations between acute measurements of MPS and muscle hypertrophy over 3 Brook et al. Taken together, these data indicate the relationship between acute measurements of MPS and chronic changes in the muscle growth response becomes apparent as the training status of the individual progresses Table 1.
The predictive value of the acute response of MPS to nutrition and exercise interventions seems to be greater in trained than untrained individuals, who are not accustomed to muscle loading during REx Damas et al.
Thus, the researcher or practitioner may wish to consider the relative value of acute measurements of MPS for predicting chronic changes in muscle growth when formulating training and nutrition recommendations, at least for trained individuals.
Relationship Between Acute Measurements of MPS and Chronic Changes in Muscle Mass in Response to RET. One physiological mechanism proposed to explain the temporal relationship between acute measurements of MPS in response to REx and chronic changes in the muscle growth response to RET relates to the nature of the response of MPS to REx Damas et al.
Damas et al. This trend aligned with the acute 48 hr muscle damage response to REx that was highest after the initial unaccustomed REx bout, but was attenuated by the early Week 3 phase of RET.
The authors reasoned that during the early phase of a training program, the increased response of MPS to REx and protein ingestion is related more to the repair and remodeling of existing older, perhaps damaged, proteins Damas, Phillips, Lixandrao, et al.
Consistent with this notion, the greater muscle damage response to unaccustomed eccentric-based exercise versus a work-matched bout of concentric exercise has been shown to correspond with a greater acute response of MPS to eccentric REx Moore et al.
As RET progresses, the responses of MPS to REx and nutrition become more refined toward muscle hypertrophy. This notion is supported by data showing that both mitochondrial and myofibrillar—MPS are increased following a REx in the untrained state Wilkinson et al. However, following 10 weeks of RET, only myofibrillar FSR is increased.
Taken together, these data suggest that with the progression of RET, and as the degree of exercise-induced muscle damage starts to diminish, the acute stimulation of MPS is directed almost exclusively to the accretion of new muscle proteins, thus explaining the correlation between acute rates of MPS and the muscle growth response during the later phase of RET Trommelen et al.
The inherent variability in the response of MPS to REx and nutrition, as well as the response of muscle hypertrophy to RET, also contributes to our inability to utilize acute metabolic data to predict an individual response to RET Figure 1. This variability in response to exercise and nutrition is reported consistently Jackman et al.
While the source of this individual variability is not fully understood at this time, genetic variability must be a contributing factor Clarkson et al. Attempts to control prestudy activity and diet are common in these studies, yet the variability is evident.
Moreover, in many studies, the population from which participants are selected is kept fairly tight. Yet, even when the range of muscle mass is restricted, there is considerable variation in the response of MPS Macnaughton et al.
The methodological conditions under which MPS is determined that may influence the measured response will be discussed below. However, in the examples illustrated in Figure 1 , the method used to determine MPS, as well as the conditions under which it was measured, in each individual were identical within studies.
Hence, methodological issues alone do not account for all the observed variability. Inherent variability in the metabolic response to REx and nutrition contributes to uncertainty in predicting muscle growth based on measured rates of MPS in individuals.
a Individual fasted FSR at rest REST and with ingestion of 30 g protein following resistance exercise FEDEX in two groups of trained young weightlifters and b individual FSR in response to ingestion of 20 and 40 g whey protein following REx in trained young weightlifters.
a Adapted from McGlory et al. Citation: International Journal of Sport Nutrition and Exercise Metabolism 32, 1; One potential contributing factor to the variability of the response of MPS to identical REx and protein feeding conditions Figure 1 might be differences in translational capacity, that is, the total number of ribosomes capable of producing peptide chains Wen et al.
The MPS is the metabolic process from which functional proteins are produced from polypeptide chains created by ribosomes. The measurement of FSR essentially represents translational efficiency, that is, the rate of translation for a given number of ribosomes.
It is clear that ribosome number, that is, translational capacity, does not change acutely following REx Brook, Wilkinson, Mitchell, et al. Thus, translational capacity may help explain the individual variability in response of MPS to anabolic stimuli.
The lack of ability to predict long-term muscle hypertrophic responses to RET with the acute measurement of MPS does not necessarily reflect the overall worth, or lack thereof, of information obtained from acute metabolic studies.
Contributing factors to the uncertain relationship between the acute MPS response to REx and nutrition, and the muscle hypertrophic response to RET, include a lack of consistency in methods utilized, as well as inherent variability resulting from the methods used Mitchell et al.
There also is heterogeneity in the response of muscle mass to RET that contributes to this disconnect. Accordingly, there are numerous reasons to suggest that the study design and methods chosen to determine hypertrophy in RET studies contributes to this quite heterogeneous response.
A full evaluation of these methods is beyond the scope of this review, so interested readers are referred to an excellent presentation of the methodology by Haun et al.
Several factors related to study design and methods used to assess MPS must be considered when interpreting the relationship between the acute response of MPS- and RET-induced changes in muscle mass. Over the past 25—30 years, the vast majority of studies investigating the response of MPS have utilized the precursor—product method with direct incorporation of the stable isotopically labeled amino acids into muscle protein to determine FSR.
Accurate prediction of muscle hypertrophy during RET by determining FSR in response to REx and nutrition requires certain assumptions to be made and met. First, we must assume that the initial measurement of FSR is representative of every subsequent stimulation of MPS for the remainder of the RET period, that is, the responses remain unchanged throughout RET see discussion above.
Next, the measured FSR captures the true response of MPS to REx and protein ingestion. Thus, methodological choices will be critical for determining the true response of MPS. Methodological considerations influence the ability to capture the true response of MPS with measurement of the FSR in response to exercise and nutrition.
Until recently, the majority of studies measuring FSR included an infusion of a labeled amino acid and multiple muscle biopsy samples.
The FSR is reported as an hourly rate of synthesis in the time between the muscle samples. An important issue for any infusion study to determine FSR is the limited time period for incorporation of the labeled amino acid.
One critical assumption is that the time between biopsies captures the true period of stimulation of MPS. Thus, regardless of the maximal magnitude of the response, if the second muscle sample is taken before the response of MPS returns to baseline, a portion of the true response of MPS may be missed and the determined FSR would be an underestimation Figure 2.
Of course, the converse would be true if the biopsy is taken too late to capture the true response. a Infusion of [ 13 C 6 ] phenylalanine and muscle samples taken at timepoints that capture the entire true response of MPS and b infusion ends and muscle samples are taken at 0 and 4 hr, but the true response of MPS remains elevated above baseline for 6 hr, so the response is underestimated.
Another factor that contributes to a mismatch between the true response of MPS to REx is the prolonged enhancement of the utilization of amino acids from protein ingestion for MPS following a REx bout Figure 3. The REx sensitizes the muscle to the anabolic stimulation of elevated amino acid levels from protein feeding Biolo et al.
It is clear that the sensitivity of muscle to amino acids remains enhanced for at least 24 hr following the exercise Burd et al.
Thus, any protein containing meal consumed within this hr time period will result in a MPS response that is greater than that in response to a meal not preceded by REx.
An acute measurement of MPS based on an infusion of labeled amino acids and biopsies for only a few hours after exercise would not be capable of capturing the contribution to muscle hypertrophy resulting from all of these enhanced postprandial elevations of MPS Figure 3a.
Thus, an acute measurement limited to only a few hours after REx would not reflect the entire influence of the exercise on MPS and subsequent muscle hypertrophy further contributing to the observed mismatch between measurement of MPS and changes in muscle mass with training.
The response of MPS is enhanced following REx and this is captured by D 2 O measurement of MPS. Over the past 15 years, another method has been revisited to determine an integrated FSR in free-living participants over a time period that is not limited by an infusion, that is, the D 2 O method Figure 3.
Thus, MPS in various situations and in response to various exercise and nutrition interventions can be determined over the time course of days to weeks. The determined rate of MPS integrates the response to all physical activity and nutrient consumption during that time, including the prolonged response of MPS to subsequent meals following REx Figure 3b.
Thus, the D 2 O method could be argued to provide a more holistic assessment of MPS without the limitations inherent with the requirement for infusion of stable isotopes for measurement of MPS. It is perhaps not particularly surprising that integrated rates of MPS over longer time periods than are possible with isotope infusion studies, as well as inclusion of habitual physical activity and enhanced periods of postprandial MPS in response to exercise hours to days earlier, are better correlated with subsequent muscle hypertrophy.
Several studies utilizing the D 2 O measurement of FSR have reported correlations of MPS with subsequent muscle hypertrophy Brook et al.
Therefore, this method for assessing MPS seems to be more suitable for predicting muscle hypertrophy with RET. The disconnect between the initial measurement of MPS and subsequent muscle hypertrophy during RET may be due to methodological choices made for measurement of changes in muscle mass in addition to MPS.
Differences in study design and methods chosen to determine changes in muscle mass, in addition to inherent individual variability in the response of muscle to training Mobley et al. Factors including training duration, sleep quality, nontraining physical activity, nutrition, and other lifestyle variables may impact the training response Haun et al.
Proper control of many of these factors is virtually impossible in most RET study situations. This variability is further complicated by the various permutations possible with various combinations of these factors Haun et al.
Perhaps a more prosaic factor contributing to the disconnect between the acute response of MPS and subsequent muscle hypertrophy with RET relates to the inherent limitations of methods used to measure changes in muscle mass in humans.
Reported changes in muscle mass with RET are heavily dependent on the method chosen to assess those changes. Hence, the critical reader should consider the limitations of these methods when evaluating any particular training study. Changes in muscle mass may be measured on one or more of several levels, that is, biochemical, ultrastructural, histological, and gross anatomical levels.
When multiple methods from these levels of hypertrophy are used, the agreement between methods is often poor Haun et al.
Moreover, as detailed above, there are different types of hypertrophy that must be considered in combination with the method chosen to assess changes in muscle mass. Three types of hypertrophy have been proposed: connective tissue, sarcoplasmic, and myofibrillar.
For example, there is evidence that hypertrophy measured at the early stage of a RET program may result from edema-induced, that is, muscle swelling and sarcoplasmic hypertrophy Damas, Phillips, Libardi, et al.
This means that if muscle hypertrophy is based on dual-energy X-ray absorptiometry or other methods without consideration of changes in intramuscular fluid, overestimations of true hypertrophy will be made.
Clearly, changes in muscle mass with fluid infiltration are not related to MPS. These methodological factors should be considered when assessing the relationship between the acute response of MPS to changes in muscle mass with RET. Based on our critical evaluation of existing evidence, we can make three practical implications.
In this review, we have attempted to provide an evidence-based critical evaluation for the use of results from acute metabolic studies to predict changes in muscle mass with RET. This lack of predictive power is especially true if the individual is beginning an unaccustomed exercise program. Nevertheless, this discrepancy should not be used to determine the value of studies measuring MPS in response to REx and protein nutrition.
There are multiple examples of studies in which the acute response of MPS does predict the average hypertrophy on a group level Hartman et al. Moreover, measurement of the acute response of MPS to REx and nutrition interventions can provide valuable information.
Regardless of training status, the acute response of MPS is indicative of protein turnover and muscle remodeling critical for recovery from exercise and adaptation to training. The measurement of integrated MPS that includes the enhanced postprandial response of MPS to protein ingestion in free-living individuals certainly may provide predictive information about subsequent muscle growth, albeit not in individuals undergoing unaccustomed exercise.
Moreover, the acute measurement of MPS also provides more sensitivity than chronic training studies over a much shorter time frame and can thus be viewed as a good starting point for determining nutritional recommendations.
Given the nature of measurement of FSR, if a difference is detected in an acute study, for example, between different protein sources, then we can conclude with high confidence that the measured difference is physiologically relevant, at least qualitatively.
In this regard, the protein source that engenders the greater FSR may be considered the higher quality protein source irrespective of whether chronic studies are able to detect differences in muscle hypertrophy under comparable conditions of protein source manipulation.
Thus, we can use that information to inform subsequent RET studies. Finally, the acute measurement of MPS in response to exercise and nutrition offers valuable mechanistic information.
In fact, delineation of mechanisms of muscle protein metabolism was the aim of many of the seminal studies that are now used to contribute to the development of recommendations Biolo et al.
Thus, whereas practitioners should be aware of the potential pitfalls with reliance on acute metabolic studies for making nutritional recommendations for athletes and exercisers, with proper interpretation a great deal of valuable information may be gleaned from these studies.
Acute measurement of MPS in response to various nutrition and exercise interventions should be viewed as yet another tool in the toolbox for use by practitioners and others. Balagopal , P. Skeletal muscle myosin heavy-chain synthesis rate in healthy humans. American Journal of Physiology, 1 , Biolo , G.
Increased rates of muscle protein turnover and amino acid transport after resistance exercise in humans. American Journal of Physiology, , E — E An abundant supply of amino acids enhances the metabolic effect of exercise on muscle protein.
Brook , M. We have shown that lipid infusion reduces the MPS response to the ingestion of amino acids during hyperinsulinemic-euglycemic clamped conditions in healthy younger adults at rest [ 41 ].
In addition, attenuated post-exercise intramuscular anabolic signaling i. The impact of the fat content of a protein-containing meal on the MPS response remains ambiguous.
Recent work suggests that the MPS response to feeding may be modulated by the consumption of micronutrients Fig. In support, the ingestion of whole eggs was more effective in stimulating post-exercise MPS rates than was the ingestion of an isonitrogenous amount of egg whites [ 40 ]. The differential response could not be attributed to differences in protein digestion and amino acid absorption or caloric intake between the treatments [ 31 , 32 , 38 ].
As previously discussed, fat co-ingestion may augment the post-exercise MPS response to protein ingestion [ 37 ]. Furthermore, several micronutrients that are contained primarily in the yolk, such as vitamin A, vitamin D, vitamin E, zinc, selenium, and cholesterol, are potential candidates to augment the anabolic response to feeding [ 30 , 43 ].
However, it should be noted that some nutrients may potentially impair MPS when ingested in high amounts that are typically not found in whole foods. For example, the ingestion of the lipid second messenger phosphatidic acid has recently been shown to impair the post-exercise MPS response in older adults [ 45 ].
Furthermore, high-dose antioxidant supplementation i. Therefore, high doses of micronutrient supplements with strong antioxidant properties is not recommended for athletes. Taken together, emerging evidence suggests that certain micronutrients in a meal may be able to modulate postprandial MPS rates.
To date, only one study has assessed postprandial MPS rates following mixed meal ingestion. Postprandial MPS rates did not differ between the ingestion of the moderate- or the high-protein meal at rest or during post-exercise recovery.
Interestingly, plasma essential amino acid concentrations were highest at the end of the 4-h postprandial period. This may suggest that large protein-rich mixed meals result in a protein digestion and absorption pattern that reflects a more slowly digestible protein.
Clearly, more research is warranted to assess the MPS response to the ingestion of mixed meals and its modulation by meal composition.
Despite warnings from health agencies, alcohol consumption remains culturally engrained worldwide [ 47 ]. Interestingly, several studies have reported that athletes are more likely to consume excessive amounts of alcohol, especially as part of binge-drinking practices in team sports [ 48 , 49 ].
Parr et al. A total of 1. These data provide clear proof of principle that ingestion of excessive amounts of alcohol can impair post-exercise recovery. Furthermore, Parr et al. How this alcohol-induced attenuation of anabolic signaling is regulated is unclear, but direct effects via REDD regulated in development and DNA damage -1 and indirect effects via the modulation of the activity of circulating anabolic hormones such as insulin-like growth factor 1 have been proposed [ 50 ].
Further work is required to provide more mechanistic insight and to determine whether there is a dose—response relationship between alcohol intake and MPS rates and to determine the impact of more moderate alcohol consumption, e. The MPS response to feeding is not only modulated by acute nutrient intake but may also be affected by habitual food intake Fig.
Athletes may intentionally eat a caloric surplus to gain lean body mass i. It is more common for athletes to purposely restrict caloric intake to reduce body fat [ 51 ].
While it is feasible to increase lean body mass during a marked energy deficit when a high protein consumption is combined with a high volume of resistance and anaerobic exercise [ 52 ], energy restriction generally leads to muscle mass loss [ 53 , 54 ].
Consistent with this notion, integrated MPS rates are reduced during energy restriction but can be rescued by resistance-type exercise and may be potentiated by a higher protein diet [ 55 ].
While acute caloric intake does not seem to modulate the MPS response to feeding [ 31 , 38 ], both acute postabsorptive and postprandial MPS rates at rest have been shown to be attenuated following a short-term 3—14 day energy intake restriction [ 10 , 56 ]. Greater reductions in the MPS response to feeding can be expected during more severe energy deficits and in individuals with lower body fat levels, as these conditions have been shown to increase lean body mass loss during energy intake restriction [ 57 , 58 ].
The blunted MPS response to protein ingestion during energy restriction may be rescued by the consumption of a higher protein diet.
Subjects consuming 1. In contrast, the opposite pattern was observed in these subjects following 10 days of weight maintenance. A significant increase in MPS was observed in subjects ingesting 0.
We observed no impact of day habituation to a higher 1. Furthermore, postabsorptive MPS rates do not appear to change even after a prolonged week high 2. While it is clear that prolonged energy restriction attenuates postabsorptive and postprandial MPS rates, the impact of habitual protein intake during energy restriction and energy balance on the MPS response to protein ingestion remains poorly understood.
Schematic representation of factors modulating the muscle protein synthetic MPS response to feeding. The MPS response to feeding is augmented by prior exercise and a higher amount, essential amino acid EEA content, and digestion and absorption rate of the ingested protein.
The MPS response to feeding is attenuated by alcohol co-ingestion, during prolonged energy deficit, during muscle inactivity, with aging especially in females , and by individuals with excessive fat mass.
indicates the impact on MPS response to feeding is unclear. Omega-3 polyunsaturated fatty acid supplementation for 8 weeks has been shown to augment the MPS response to a hyperinsulinemic—hyperaminoacidic clamp in both younger and older adults [ 11 , 61 ]. As postabsorptive MPS rates were not significantly increased following the supplementation period, omega-3 supplementation appears to specifically enhance the muscle anabolic sensitivity to hyperaminoacidemia.
Insulin and amino acids were clamped to levels typically seen after a meal but were low enough to avoid a possible ceiling effect. In contrast, omega-3 supplementation for 8 weeks did not increase the MPS response to the ingestion of 30 g of whey protein at rest or following resistance-type exercise [ 62 ].
As 30 g of protein should be more than sufficient to maximize the MPS response to protein ingestion in healthy young subjects, it could be speculated that fish oil supplementation may only augment the MPS response to suboptimal amounts of protein ingestion. While omega-3 fatty acid supplementation could provide an effective nutritional strategy to augment the MPS response to protein ingestion, it is unclear what the target omega-3 concentrations should be and thus what dose and duration of supplementation period should be recommended.
Physical activity and exercise are potent stimulators of postabsorptive as well as postprandial MPS rates [ 2 , 63 , 64 , 65 ] Fig.
A single bout of exercise stimulates the use of dietary protein-derived amino acids as precursors for MPS, as dietary protein-derived amino acids are more directed towards activated muscle during their recovery from exercise [ 66 ]. The MPS response to protein ingestion is maximally stimulated by the ingestion of 20 g of whey protein in rested conditions and following lower-body resistance-type exercise [ 4 , 5 ], albeit with higher MPS rates during the post-exercise condition [ 4 , 5 ].
However, exercise may increase not only the maximal rate of postprandial MPS but also the duration of the anabolic response. However, the exercise-induced increase in anabolic sensitivity may supplant the muscle full effect and allow for a more prolonged MPS response to feeding.
In support, two studies using a unilateral leg exercise model observed a prolonged MPS response to feeding in the exercised leg [ 68 , 69 ]. MPS rates were elevated in the first 3 h following protein ingestion but had returned to basal levels in the subsequent 2 h in the rested leg, whereas MPS rates remained elevated during the entire 5-h period following protein ingestion in the exercised leg [ 68 , 69 ].
Thus, exercise appears to increase both the rate and the duration of postprandial MPS rates. MPS rates are also sensitive to habitual physical activity.
Several studies have demonstrated that muscle disuse reduces postabsorptive and postprandial MPS rates following prolonged bed rest or immobilization [ 70 , 71 , 72 , 73 ].
Glover et al. As the higher amino acid infusion protocol provided ample amino acids to maximize the MPS response under normal conditions, it appears that hyperaminoacidemia cannot compensate for a disuse-induced decline in muscle anabolic sensitivity.
Less extreme reductions in physical activity may also reduce anabolic sensitivity in muscle. For example, a reduction in daily step count has also been shown to reduce the MPS response to feeding in older adults [ 72 ].
To date, the impact of step reduction on MPS rates in healthy younger adults has not been assessed. It could be speculated that a reduction in daily step count has less impact on anabolic sensitivity in athletes undergoing intensive exercise training, as low-load resistance-type exercise has been shown to attenuate the decline in anabolic sensitivity during step reduction in older adults [ 71 ].
However, the MPS response to feeding is likely reduced during prolonged bed rest or immobilization and may contribute to injury-related muscle atrophy in athletes [ 74 ]. An injured athlete is likely to have a lower exercised-induced energy expenditure and is likely to reduce caloric intake to avoid gaining body fat.
However, a reduction in energy intake may result in a lower absolute protein intake. While the limited work available does not support the idea that a higher protein intake can compensate for a disuse-induced reduction in MPS, habitual protein should at least be maintained [ 74 , 75 ].
Ageing is accompanied by a progressive decline in muscle mass, termed sarcopenia. Little to no differences in postabsorptive MPS rates are observed between younger and older adults [ 76 , 77 ]. However, the MPS response to feeding is attenuated in older compared with younger adults, a phenomenon termed anabolic resistance [ 77 ].
Interestingly, it appears the age-related anabolic resistance to protein ingestion can be at least partly compensated for by increasing the amount of ingested protein. Therefore, possibly even greater amounts of ingested protein are required to maximize the MPS response to feeding in older adults.
Alternatively, it is not clear whether 40 g of dietary protein is required to maximize the anabolic response in older adults or whether a more moderate dose such as 30 g is sufficient. The average weight of the older adults in this analysis was Therefore, older athletes should aim to ingest at least 30 g of high-quality protein per meal to improve exercise recovery and adaptations.
Men have more muscle mass and less body fat than age- and bodyweight-matched females [ 80 ]. However, in healthy young adults, no such sexual dimorphism is apparent in basal MPS rates [ 11 , 81 , 82 , 83 ], MPS response to a hyperinsulinemic—hyperaminoacidemic clamp [ 11 , 83 ], MPS response to resistance-type exercise [ 82 ], or postprandial MPS rates following resistance-type exercise [ 81 ].
A possible explanation for this apparent discrepancy is that the greater amount of muscle mass in males than in females primarily originates from an augmented growth spurt in males during puberty, which seems attributable to the surge in testosterone secretion [ 13 , 84 ].
After puberty, muscle mass remains largely constant up to middle-age adulthood in both healthy males and healthy females, which is consistent with similar muscle protein turnover rates between the sexes.
Therefore, nutritional strategies to maximize the muscle anabolic response do not differ between young male and female adults per se.
In contrast to younger adults, a sexual dimorphism in MPS rates may exist in adults at a more advanced age.
Older women have higher postabsorptive MPS rates but a blunted MPS response to feeding compared with older men at rest [ 13 , 83 , 85 ]. As greater amounts of protein in a meal can at least partly compensate for age-related anabolic resistance [ 78 ], it is tempting to speculate that older women require the ingestion of higher amounts of protein to maximize the anabolic response to feeding when compared with older men.
In addition, whether age-related sexual dimorphism in the MPS response to protein feeding is present in active older adults engaging in regular exercise training remains to be determined.
It seems intuitive that individuals with a greater lean body mass require larger amounts of protein to be consumed than do individuals with less lean body mass. Consistent with this line of thinking, protein intake requirements are often expressed relative to body size, and most typically to body weight, as this is more practical to assess in individuals.
However, only one study has directly examined the impact of body size on the MPS response to feeding. Macnaughton et al. These data suggest that lean body mass is not a strong modulator of protein requirements in the initial several hours of post-exercise recovery.
A possible explanation is that only a relatively small amount of essential amino acids is required as precursors for MPS, even in larger individuals. A factor that is more likely to limit the MPS response to feeding is the postprandial rise in plasma leucine concentration [ 68 ].
However, Macnaughton et al. Therefore, the amount of ingested protein required to maximize the muscle anabolic response following resistance-type exercise may be less affected by body size than has been assumed. What may cause a reduced MPS response to feeding in subjects with excess body fat is unclear, but it seems unlikely that the amount of body fat an individual possesses has a substantial impact on the amount of precursors required for MPS or the size of the plasma amino acid pool and consequently the peak plasma leucine concentrations following feeding.
Therefore, it is more likely that excess body fat directly reduces anabolic sensitivity to protein ingestion in muscle. In support, lipid infusion has been shown to reduce postprandial MPS rates in healthy young adults at rest [ 41 ]. These data suggest that excess lipid availability per se reduces anabolic sensitivity within skeletal muscle, independent of body composition [ 41 ].
Therefore, athletes who are intentionally overfeeding to gain muscle mass may consider limiting excessive dietary fat intake and body fat accumulation. However, the proposed impact of excessive body fat mass on MPS rates is likely confounded by habitual physical activity levels and is perhaps less of a concern for athletes engaged in regular intense exercise training.
It could be argued that the anabolic response to feeding is not limited to the MPS response. Muscle protein net balance is determined by the difference between MPS and muscle protein breakdown rates.
However, changes in MPS rates in response to exercise and nutrition seem to be much greater than changes observed in muscle protein breakdown rates [ 2 , 90 ]. Therefore, changes in net muscle protein balance appear to be largely determined by changes in MPS rates.
While feeding reduces muscle protein breakdown rate via an increase in circulating plasma insulin concentrations, only a moderate rise in insulin concentration is required for maximal inhibition of muscle breakdown rates.
Greenhaff et al. However, it appears even lower insulin concentrations may be sufficient to suppress post-exercise muscle protein breakdown rates.
Therefore, food intake will substantially reduce muscle protein breakdown rates, with the macronutrient content of the food being of little impact. Although under normal, healthy conditions, protein breakdown does not seem to play an important quantitative role in net muscle accretion, it does have an important function in muscle tissue reconditioning.
In support, knocking out critical genes in the protein breakdown proteasome and autophagy pathways reduces muscle quality, muscle function, and muscle mass in animal models [ 91 , 92 , 93 , 94 ]. These data suggest that at least some amount of muscle protein breakdown is required for proper muscle conditioning.
Therefore, inhibition of muscle protein breakdown beyond the normal postprandial reduction may not represent a desirable target in healthy populations.
To avoid skeletal muscle tissue sampling, protein synthesis and protein breakdown rates are often assessed on a whole-body level, which only requires stable isotope tracer infusion and arterial or arterialized blood sampling [ 95 ].
However, muscle tissue has a relatively slow turnover when compared with other tissues, such as liver, kidney, lung, intestine [ 96 ], and even brain [ 97 ]. As a consequence, whole-body and MPS rates in response to nutrition [ 27 , 34 , 40 ], exercise [ 65 , 99 ], and disease [ , ] do not necessarily align.
Consequently, we should be cautious when applying whole-body amino acid kinetics to gain insight into protein metabolism at a tissue-specific level.
Practical inferences based on the assessment of whole-body protein metabolism are further complicated by methodological issues. A common method to assess whole-body protein metabolism is based on the amino acid flux in and out of the circulation.
This method assumes that the rate at which amino acids are disappearing from the circulation i. This would require the tissue free amino acid pools to remain constant.
However, tissue free amino acid pools are likely to change considerably in response to conditions such as feeding, invalidating whole-body protein synthesis rate calculations. Even more complex is the determination of whole-body protein breakdown rates in a postprandial setting.
Whole-body protein breakdown rates are determined by the rate at which amino acids are appearing in the circulation from tissues minus the rate at which dietary protein-derived amino acids appear in the circulation. Therefore, calculation of whole-body protein breakdown rates depends on accurate assessment of the amount of exogenous protein appearing in the circulation.
Some researchers estimate the latter based on previously published values [ 34 , , ]. However, such values are specific to the experimental conditions under which they are obtained i. Therefore, assessment of postprandial whole-body protein breakdown rates is only reliable when the amount of exogenous protein appearing in the circulation is assessed appropriately [ ].
The indicator amino acid technique is a noninvasive method to assess whole-body protein balance [ ]. In contrast, the ingestion of 20—40 g of high-quality protein is sufficient to maximize the postprandial MPS response in healthy young adults at rest or following resistance-type exercise [ 4 , 5 , 17 ].
However, clearly more research is warranted to determine which non-muscle tissues are responsive to protein feeding and whether this has any functional relevance for athletes.
Whether changes in MPS rates following certain stimuli correlate with subsequent changes in muscle mass during more prolonged exposure to such stimuli has been questioned.
Mitchell et al. Myofibrillar protein synthesis rates were assessed in the first 6 h following the first exercise bout performed as part of the prolonged exercise training program. The lack of significant positive correlations is not surprising as basal and postprandial myofibrillar protein synthesis rates have been shown to be elevated for up to 72 h after a single bout of exercise [ 63 ].
A follow-up study assessed myofibrillar protein synthesis rates during a more prolonged h post-exercise period after the initial exercise bout, at 3 weeks, and at 10 weeks of a prolonged resistance-type exercise training program [ ].
While no correlations were observed between post-exercise myofibrillar protein synthesis rates following the initial exercise bout and muscle hypertrophy following prolonged exercise training, strong positive correlations were observed between myofibrillar protein synthesis rates assessed over a h period at 3 weeks and at 10 weeks of training and the increase in muscle mass.
The initial exercise bout resulted in considerable muscle damage, but exercise-induced muscle damage was attenuated at 3 weeks of training and almost completely absent at 10 weeks. Therefore, it appears that the myofibrillar protein synthetic response to a single bout of unaccustomed exercise may be at least partly a response to muscle damage and directed at tissue repair rather than muscle hypertrophy.
After the first couple of days or weeks of training, post-exercise myofibrillar protein synthesis rates may be more reflective of the net changes in muscle mass, i. Consistent with this notion, myofibrillar protein synthesis rates assessed during several weeks of a prolonged resistance-type training program have been shown to correlate with muscle hypertrophy [ ].
Given these findings, it seems evident that changes in myofibrillar protein synthesis rates during recovery from successive exercise sessions can be predictive of net increase in muscle mass.
The ingestion of 20 g of high-quality, rapidly digestible protein results in a near-maximal stimulation of MPS rates at rest and during the initial several hours of recovery following lower-body resistance-type exercise.
Ingestion of animal-derived proteins tends to result in a greater increase in MPS rates than ingestion of plant-derived proteins. Recent evidence suggests that whole-food protein sources may contain micronutrients that can further augment the MPS response. The anabolic response to protein ingestion is attenuated during prolonged energy intake restriction, during muscle disuse, and in older adults especially older females.
The ingestion of greater amounts of protein can at least partly rescue the blunted MPS response during prolonged energy restriction and ageing but not during muscle disuse. In conclusion, nutritional recommendations to maximize the MPS response to feeding depend on both the type of meal and time until the next feeding opportunity and should be personalized to the individual athlete.
Biolo G, Maggi SP, Williams BD, Tipton KD, Wolfe RR. Increased rates of muscle protein turnover and amino acid transport after resistance exercise in humans. Am J Physiol. CAS PubMed Google Scholar. Phillips SM, Tipton KD, Aarsland A, Wolf SE, Wolfe RR.
Mixed muscle protein synthesis and breakdown after resistance exercise in humans. Tipton KD, Ferrando AA, Phillips SM, Doyle DJ, Wolfe RR. Postexercise net protein synthesis in human muscle from orally administered amino acids.
Article CAS PubMed Google Scholar. Moore DR, Robinson MJ, Fry JL, Tang JE, Glover EI, Wilkinson SB, et al. Ingested protein dose response of muscle and albumin protein synthesis after resistance exercise in young men.
Am J Clin Nutr. Witard OC, Jackman SR, Breen L, Smith K, Selby A, Tipton KD. Myofibrillar muscle protein synthesis rates subsequent to a meal in response to increasing doses of whey protein at rest and after resistance exercise.
Tang JE, Moore DR, Kujbida GW, Tarnopolsky MA, Phillips SM. Ingestion of whey hydrolysate, casein, or soy protein isolate: effects on mixed muscle protein synthesis at rest and following resistance exercise in young men.
J Appl Physiol. Google Scholar. Wilkinson SB, Tarnopolsky MA, Macdonald MJ, Macdonald JR, Armstrong D, Phillips SM. Consumption of fluid skim milk promotes greater muscle protein accretion after resistance exercise than does consumption of an isonitrogenous and isoenergetic soy-protein beverage.
Levenhagen DK, Gresham JD, Carlson MG, Maron DJ, Borel MJ, Flakoll PJ. Postexercise nutrient intake timing in humans is critical to recovery of leg glucose and protein homeostasis.
Am J Physiol Endocrinol Metab. Gorissen SHM, Remond D, van Loon LJC. The muscle protein synthetic response to food ingestion. Meat Sci. Hector AJ, Marcotte GR, Churchward-Venne TA, Murphy CH, Breen L, Von Allmen M, et al.
Whey protein supplementation preserves postprandial myofibrillar protein synthesis during short-term energy restriction in overweight and obese adults. J Nutr. Smith GI, Atherton P, Reeds DN, Mohammed BS, Rankin D, Rennie MJ, et al.
Omega-3 polyunsaturated fatty acids augment the muscle protein anabolic response to hyperinsulinaemia—hyperaminoacidaemia in healthy young and middle-aged men and women. Clin Sci Lond. Article CAS PubMed PubMed Central Google Scholar. Beals JW, Sukiennik RA, Nallabelli J, Emmons RS, van Vliet S, Young JR, et al.
Anabolic sensitivity of postprandial muscle protein synthesis to the ingestion of a protein-dense food is reduced in overweight and obese young adults. Smith GI, Mittendorfer B. Sexual dimorphism in skeletal muscle protein turnover. Moore DR, Camera DM, Areta JL, Hawley JA.
Beyond muscle hypertrophy: why dietary protein is important for endurance athletes 1. Appl Physiol Nutr Metab. Parr EB, Camera DM, Areta JL, Burke LM, Phillips SM, Hawley JA, et al. Alcohol ingestion impairs maximal post-exercise rates of myofibrillar protein synthesis following a single bout of concurrent training.
PLoS One. Pasiakos SM, McClung HL, Margolis LM, Murphy NE, Lin GG, Hydren JR, et al. Human muscle protein synthetic responses during weight-bearing and non-weight-bearing exercise: a comparative study of exercise modes and recovery nutrition.
Macnaughton LS, Wardle SL, Witard OC, McGlory C, Hamilton DL, Jeromson S, et al. The response of muscle protein synthesis following whole-body resistance exercise is greater following 40 g than 20 g of ingested whey protein.
Physiol Rep ;4 van Vliet S, Burd NA, van Loon LJ. The skeletal muscle anabolic response to plant- versus animal-based protein consumption.
Article PubMed Google Scholar. Gorissen SH, Horstman AM, Franssen R, Crombag JJ, Langer H, Bierau J, et al. Ingestion of wheat protein increases in vivo muscle protein synthesis rates in healthy older men in a randomized trial.
Reidy PT, Walker DK, Dickinson JM, Gundermann DM, Drummond MJ, Timmerman KL, et al. Protein blend ingestion following resistance exercise promotes human muscle protein synthesis.
Pennings B, Boirie Y, Senden JMG, Gijsen AP, Kuipers H, van Loon LJC. Whey protein stimulates postprandial muscle protein accretion more effectively than do casein and casein hydrolysate in older men. Burd NA, Yang Y, Moore DR, Tang JE, Tarnopolsky MA, Phillips SM.
Greater stimulation of myofibrillar protein synthesis with ingestion of whey protein isolate v. micellar casein at rest and after resistance exercise in elderly men. Br J Nutr. Koopman R, Crombach N, Gijsen AP, Walrand S, Fauquant J, Kies AK, et al. Ingestion of a protein hydrolysate is accompanied by an accelerated in vivo digestion and absorption rate when compared with its intact protein.
Wall BT, Hamer HM, de Lange A, Kiskini A, Groen BBL, Senden JMG, et al. Leucine co-ingestion improves post-prandial muscle protein accretion in elderly men.
com cannot guarantee each citation it generates. Sports Sports fitness recreation and leisure magazines Muscle Protein Synthesis. Muscle Protein Synthesis gale. MLA Chicago APA " Muscle Protein Synthesis. Learn more about citation styles Citation styles Encyclopedia.
More From encyclopedia. com Hydration , Hydration is the process by which water is ingested and absorbed into the body. Given the essential role that water plays in so many bodily processes… Phosphocreatine , Phosphocreatine is a substance that, in its chemical partnership with adenosine triphosphate ATP , is fundamental to the ability of the body to prod… Cramp , cramp Muscle cramps are one of the most common clinical problems suffered by athletes in endurance events.
A third to a half of marathon runners and… Sports Injuries , The treatment and management of sports injuries has become a multi-faceted and highly visible aspect of sports science. Sports medicine is a distinct… Ephedra , Ephedra, a short form for the scientific name ephedra sinica, is also known as ma huang, Mormon tea, and other descriptions.
Ephedra leaves have been… Carbohydrate , Carbohydrates are the fuel with which the body gains energy. Carbohydrates are the most prominent example of a substance that has a wide name recogni….
About this article Muscle Protein Synthesis Updated About encyclopedia. com content Print Article. You Might Also Like Protein Ingestion and Recovery from Exercise. Physiology of Exercise. Exercise Recovery.
Whole-Body Heat Cramping. Exercise and Fluid Replacement. Salt Tablets. Sport Nutrition. NEARBY TERMS Muscle Mass and Strength. Muscle Glycogen Recovery.
Muscle Fibers: Fast and Slow Twitch. Muscle Cramps. Muscle Contraction. Muscle Cars. Muscle Beach. Muscatine, Doris
Maximizing synthfsis post-exercise increase Protein and muscle protein synthesis in athletes muscle protein RPotein, especially of athletea contractile myofibrillar protein fraction, is essential to facilitate effective muscle remodeling, and enhance hypertrophic gains with resistance training. MPS is the primary regulated variable influencing muscle net balance with dietary amino acid ingestion representing the single most important nutritional variable enhancing post-exercise rates of muscle protein synthesis. Dose-response studies in average i. Re-analysis of published literature in young adults suggests a relative single meal intake of ~0. This muscle-specific bolus intake is lower than that reported to maximize whole body anabolism i. Muscle protein synthesis is essential for exercise recovery anc adaptation. But Improve blood pressure levels znd used to measure muscle protein synthesis in studies are Antiviral immune-boosting herbs complicated. The purpose of this article is Exotic coffee beans provide a comprehensive guide on Exotic coffee beans protein synthesis: syntyesis is athlrtes, how is it measured, what are the strengths and limitations, how to draw proper conclusions from muscle protein synthesis research, and of course practical guidelines how to optimize it. Please note that section 3 described the various methods to measure muscle protein synthesis. You might want to skip this section if you just want exercise and nutrition guidelines to optimize gains. Perhaps you can come back to it later when you are ready to become a true muscle protein synthesis research master.
0 thoughts on “Protein and muscle protein synthesis in athletes”