Ribose sugar and cell growth -
Yang, S. Pancreatic cancers require autophagy for tumor growth. Zhao, H. Tumor microenvironment derived exosomes pleiotropically modulate cancer cell metabolism. eLife 5 , e Macrophage-released pyrimidines inhibit gemcitabine therapy in pancreatic cancer. Cell Metab. e6 Sousa, C. Pancreatic stellate cells support tumour metabolism through autophagic alanine secretion.
Kim, P. Hyaluronic acid fuels pancreatic cancer cell growth. eLife 10 , e Klijn, C. A comprehensive transcriptional portrait of human cancer cell lines. Possemato, R.
Functional genomics reveal that the serine synthesis pathway is essential in breast cancer. Barretina, J.
The Cancer Cell Line Encyclopedia enables predictive modelling of anticancer drug sensitivity. Choi, J. Uridine protects cortical neurons from glucose deprivation-induced death: possible role of uridine phosphorylase.
Neurotrauma 25 , — Uridine prevents the glucose deprivation-induced death of immunostimulated astrocytes via the action of uridine phosphorylase. Wice, B. The continuous growth of vertebrate cells in the absence of sugar. Geiger, A. Cytidine and uridine requirement of the brain. Löffler, M.
Cytokinetic studies on the switch from glucose to uridine metabolism, and vice versa, of Ehrlich ascites tumour cells in vitro. Cell Prolif. Article Google Scholar. Linker, W. Uridine, but not cytidine can sustain growth of Ehrlich ascites tumor cells in glucose-deprived medium with altered proliferation kinetics.
Cell Biol. CAS PubMed Google Scholar. King, M. Human cells lacking mtDNA: repopulation with exogenous mitochondria by complementation. Science , — Article CAS PubMed ADS Google Scholar. Son, J. Glutamine supports pancreatic cancer growth through a KRAS-regulated metabolic pathway.
Lee, H. A large-scale analysis of targeted metabolomics data from heterogeneous biological samples provides insights into metabolite dynamics. Metabolomics 15 , Yuan, M. Sullivan, M. Quantification of microenvironmental metabolites in murine cancers reveals determinants of tumor nutrient availability.
eLife 8 , e Hezel, A. Genetics and biology of pancreatic ductal adenocarcinoma. Kerk, S. Metabolic networks in mutant KRAS-driven tumours: tissue specificities and the microenvironment.
Cancer 21 , — Collins, M. Oncogenic Kras is required for both the initiation and maintenance of pancreatic cancer in mice. Ying, H. Oncogenic Kras maintains pancreatic tumors through regulation of anabolic glucose metabolism.
Cell , — Candido, J. Cell Rep. Zhang, Y. Gut 66 , — Zhang, D. pdependent suppression of uridine phosphorylase gene expression through direct promoter interaction. Dalin, S. Deoxycytidine release from pancreatic stellate cells promotes gemcitabine resistance.
Tabata, S. Thymidine catabolism as a metabolic strategy for cancer survival. Wang, T. Jurkowitz, M. Adenosine, inosine, and guanosine protect glial cells during glucose deprivation and mitochondrial inhibition: correlation between protection and ATP preservation. Litsky, M. Inosine and guanosine preserve neuronal and glial cell viability in mouse spinal cord cultures during chemical hypoxia.
Brain Res. Salvage of ribose from uridine or RNA supports glycolysis in nutrient-limiting conditions. Puleo, F. Stratification of pancreatic ductal adenocarcinomas based on tumor and microenvironment features.
e3 Vaas, L. opm: an R package for analysing OmniLog R phenotype microarray data. Bioinformatics 29 , — Ran, F. Genome engineering using the CRISPR—Cas9 system.
Metabolic requirement for GOT2 in pancreatic cancer depends on environmental context. eLife 11 , e Yi, Z. KDM6A regulates cell plasticity and pancreatic cancer progression by noncanonical activin pathway.
Scales, M. Combinatorial Gli activity directs immune infiltration and tumor growth in pancreatic cancer. PLoS Genet. Uhlén, M. Tissue-based map of the human proteome. Science , Barrett, T. NCBI GEO: archive for functional genomics data sets—update.
Nucleic Acids Res. Goldman, M. Visualizing and interpreting cancer genomics data via the Xena platform. Tsherniak, A. Defining a cancer dependency map. Cell , — e16 Gearing, L. CiiiDER: A tool for predicting and analysing transcription factor binding sites. PLoS ONE 14 , e Steele, N.
Multimodal mapping of the tumor and peripheral blood immune landscape in human pancreatic cancer. Cancer 1 , — Download references. We thank B. Bochner for providing the OmniLog instrument, helping with the data analysis, countless insightful discussions, and support; S.
Chan for support with Biolog data collection and analysis; D. DeNardo and the DeNardo laboratory for support designing the macrophage-depletion studies; N.
Guppy for processing and immunohistochemistry staining of mouse tumour samples; L. Howell for helping with the automated image analysis of immunohistochemistry staining; and members of the Sadanandam and Lyssiotis laboratories and the entire Pancreatic Disease Initiative at the Rogel Cancer Center, University of Michigan, for their insightful comments and discussions.
was supported by the Department of Veteran Affairs Career Development Award IK2BX was supported by American Cancer Society IRG , the University of Chicago Cancer Center Support Grant P30 CA , the Pancreatic Cancer Action Network Career Development Award , the Brinson Foundation, the Cancer Research Foundation and the Ludwig Center for Metastasis Research.
and C. were supported by UMCCC Core Grant P30CA The funders had no role in study design, data collection and analysis, or the content and publication of this manuscript. These authors contributed equally: Zeribe C. Nwosu, Matthew H. Ward, Peter Sajjakulnukit, Pawan Poudel.
Department of Molecular and Integrative Physiology, University of Michigan, Ann Arbor, MI, USA. Zeribe C. Ward, Peter Sajjakulnukit, Steven Kasperek, Megan Radyk, Damien Sutton, Anthony Andren, Zachary Tolstyka, Ho-Joon Lee, Julia Ugras, Li Zhang, Christopher J.
Department of Chemistry, Washington University in St Louis, St Louis, MO, USA. Matthew H. Ward, Leah P. Department of Medicine, Washington University in St Louis, St Louis, MO, USA.
Center for Metabolomics and Isotope Tracing, Washington University in St Louis, St Louis, MO, USA. Division of Molecular Pathology, The Institute of Cancer Research, London, UK. Cellular and Molecular Biology Program, University of Michigan, Ann Arbor, MI, USA.
Ben May Department for Cancer Research, University of Chicago, Chicago, IL, USA. Juan J. Apiz-Saab, Lindsey N. Department of Surgery, University of Michigan, Ann Arbor, MI, USA. Department of Internal Medicine, Division of Gastroenterology, University of Michigan, Ann Arbor, MI, USA.
Department of Pathology and Clinical Labs, Rogel Cancer Center, University of Michigan, Ann Arbor, MI, USA. Rogel Cancer Center, University of Michigan, Ann Arbor, MI, USA. Centre for Global Oncology, Division of Molecular Pathology, The Institute of Cancer Research, London, UK.
You can also search for this author in PubMed Google Scholar. designed the study. wrote the manuscript. designed experiments, and Z. and P. collected data for the bulk of the experimental studies. prepared manuscript figures. and Z. carried out the initial cell line screen, and P. conducted the analysis of the screening data that revealed lead metabolite—enzyme interactions.
carried out experiments. and E. contributed to the data analysis. provided resources, funding and conceptual input for experiments and supervised the research. All authors reviewed and approved the final manuscript. Correspondence to Anguraj Sadanandam or Costas A. Nature thanks the anonymous reviewers for their contribution to the peer review of this work.
Peer review reports are available. Schematic overview of the parameters measured by the Biolog Phenotype Microarray. Heatmap showing the high confidence metabolites HCMs , namely, the metabolites that were utilized above or below the median of negative controls as determined by one-tailed Wilcoxon rank sum test.
Legend denotes fold change relative to median negative control signal, where red shows high utilization and blue shows low utilization.
Spearman correlations, r, between UPP1 expression in cell lines ref. On the right: GSEA plot indicating the enrichment of glycolysis hallmark in the UPP1 high relative to the low tumours.
NES, normalized enrichment score. Analysis was based on the differential genes derived from CCLE data and part of the data shown in Fig. GSEA plots of significantly enriched KEGG pathways in UPP1 -high PDA tumours relative to UPP1 low tumours.
Plots are part of the data e from the analysis of GSE human PDA dataset. Statistics and reproducibility: a , The kinetic measurement evaluated several parameters, including the time taken for cells to adapt to and catabolize a nutrient lambda , the rate of uptake and catabolism mu or slope , the total metabolic activity area under the curve; AUC , and the maximum metabolic activity.
The values from the maximum catabolic efficiency maximum height, A of the respective metabolites were used to determine relative metabolic activity RMA. Relative RMA upon uridine supplementation with or without glucose and glutamine. Plots in f-g are from the same experiment as d-e.
Statistical significance was measured using two-tailed unpaired t-test. Data a, f, g, h are shown as mean ± s. ADP, adenosine diphosphate; AMP, adenosine monophosphate; GSSG, oxidized glutathione; NADH, nicotinamide adenine dinucleotide; UDP-GlcNAc, uridine diphosphate N-acetylglucosamine; X5P, xylulose 5-phosphate.
Mode of uridine injection is intratumoural for Sub-Q and intraperitoneal for Ortho. Isotope tracing showing metabolite labelling upon supplementation with 13 C 5 -uridine at the TIF uridine and glucose concentrations shown in Fig.
AXP — AMP, ADP, ATP, and related metabolites; UXP — UMP, UDP, UTP and related metabolites. The experiments a-d were performed once.
Data a-d are shown as mean ± s. d, where applicable. Schematic depicting metabolic pathways for uridine utilization. Expression of PGM2 , UCK1 , and UCK2 in non-tumour NT and PDA tissue samples from the GSE dataset.
Expression of PGM2 , UCK1 and UCK2 in TCGA human PDA tumour and CCLE human cell line data separated into UPP1 low L and high H subsets. Western blot for PGM2 in PDA cell lines. Presented in bold are cells that express high UPP1.
These samples are the same batch as the data shown in Fig. kDa, unit for molecular weight. qPCR for PGM2 in ASPC1 cells transfected with siPGM2 compared to non-targeting siNT control.
qPCR for UCK1 in ASPC1 cells transfected with siUCK1 compared to non-targeting siNT control. qPCR for UCK2 in ASPC1 cells transfected with siUCK2 compared to non-targeting siNT control.
All four group comparisons have significant P: 0. Uridine can also be catabolized via UPP1 to produce uracil and ribose 1-phosphate. Ribose 1-phosphate is converted to ribosephosphate by PGM2 and fuel pentose phosphate pathway, nucleotide biosynthesis and glycolysis.
TCGA — The Cancer Genome Atlas, CCLE — Cancer Cell Line Encyclopaedia. Vinculin is used as a loading control. RMA — statistical significance was measured using multiple unpaired t tests with two-stage two-step method. Data a—c, f—h, j—l are shown as mean ± s.
The experiments were performed once a—c, k , and twice j,l with similar results. Mass isotopologue distribution of 1 mM 13 C 5 -uridine ribose-derived carbon into the indicated metabolic pathways in wildtype WT or UPP1-KO PATUS and ASPC1 cells.
Data a—b, d—k are shown as mean ± s. Metabolomics experiments were done once. TCGA RNA seq data showing the expression of UPP1 and its paralog UPP2. FPKM, fragments per kilobase of exon per million mapped fragments. RNA seq showing UPP1 expression in various normal human tissues Human Protein Atlas data , as obtained from the National Center for Biotechnology Information NCBI portal.
Histological data showing UPP1 protein expression in normal pancreatic tissue compared to PDA. UPP1 expression in human non-PDA cancers accessed in publicly accessible datasets. A lung cancer cell line, comparison between no uridine and 0.
RPKM, reads per kilobase of exon per million reads mapped. Data a-b, f shown as mean ± s. Statistical significance was tested using two-sided Wilcoxon or Kruskal-Wallis tests.
RNAscope images showing UPP1 expression in tumour PDA compared to the adjacent non-tumour tissues. Pan-cytokeratin PanCK indicates the tumour cells; DAPI, nuclear stain. The images are representative of three 20x acquisitions per tissue slide, and of two independent experiments.
Scale bar indicates µm. UMAP plot showing the expression of UPP1 at the transcript level, as determined by single cell RNA seq of PDA tissues from two patients and Violin plots showing UPP1 expression in various tumour microenvironment cell types, including myeloid and epithelial cells where UPP1 is highest.
Data used in plots b-c are from a previously published dataset Immunohistochemistry of UPP1 in patient biopsy sections from previously published tissue microarray Micrographs are representative from patient samples in the microarray and two independent experiments.
Kaplan-Meier plot showing survival probability log-rank test based on UPP1 expression in three separate datasets. Each dataset was split into two — UPP1 high and UPP1 low — based on the ranked UPP1 expression value.
TME — tumour microenvironment. Normalized UPP1 protein expression in Kras wildtype and mutant cell lines based on CCLE protein data accessed via the DepMap portal.
Densitometric quantification of pERK and UPP1 in the ASPC1 blots shown in Fig. MTT assay showing relative proliferation of PDA cell lines treated with 1.
Statistics and reproducibility: a , Sample size — wild type 0 and mutation 1: and 69 pan-cancer , 15 and 15 colon cancer , 54 and 25 lung cancer. Statistical significance was measured using two-tailed unpaired t test.
ERK and Vinculin are used as loading controls. Blots are representative of two biological and technical replicates for ASPC1 and one biological replicate for PATUS and DANG with similar results.
The experiments e, g, h were performed once with similar results on UPP1 displayed by the three cell lines. This blot was run on the same gel as Fig. Blots c,i are representative of two independent experiments; blot e experiment was done once. Data b, e, g-h, l shown as mean ± s. On the right: UPP1 mRNA expression determined by qPCR.
CiiDER analysis of transcription factor binding sites in the promoters of mouse and human UPP1. Myc binding sites were not detected. qPCR showing UPP1 expression upon uridine supplementation with or without basal glucose concentration in culture medium. RNA seq data showing the expression of Upp1 in sorted tumour cells and in KPC cells cultured in vitro in regular RPMI culture medium or tumour interstitial fluid medium TIFM.
Blots shown a-b are representative of two biological and technical replicate analyses with similar results. Data a,b,d,e shown as mean ± s. Data was extracted from a previously published metabolomics Relative uracil abundance in plasma, tumour interstitial fluid TIF , and bulk tumour from the experiment described in Fig.
Micrographs are representative of 10 fields per image obtained per experiment group. On the right is the respective quantification of each IHC stain.
Color scale denotes fold change. Below: Venn diagram showing overlapping metabolites that accumulated in both human PATUS and ASPC1 and mouse MTD cell lines upon UPP1 knockout. On the right: bulk tumour uridine and uracil as measured using metabolomics.
Data a, b, d, e shown as mean ± s. d; horizontal bars in h represent mean value. Source data for metabolomics, mouse studies and qPCR. Full source data for all the metabolomics and tumour studies are presented, labelled by sub-figure and separated by tabs.
Additionally, mRNA expression is provided for PGM2 , UCK1 and UCK2 following siRNA-mediated knockdown. Open Access This article is licensed under a Creative Commons Attribution 4. Reprints and permissions. Nwosu, Z. Uridine-derived ribose fuels glucose-restricted pancreatic cancer.
Download citation. Received : 21 June Accepted : 12 April Published : 17 May Issue Date : 01 June Anyone you share the following link with will be able to read this content:. Sorry, a shareable link is not currently available for this article.
Provided by the Springer Nature SharedIt content-sharing initiative. By submitting a comment you agree to abide by our Terms and Community Guidelines. If you find something abusive or that does not comply with our terms or guidelines please flag it as inappropriate.
Sign up for the Nature Briefing: Cancer newsletter — what matters in cancer research, free to your inbox weekly. Skip to main content Thank you for visiting nature.
nature articles article. Download PDF. Subjects Cancer metabolism Metabolomics Pancreatic cancer. Abstract Pancreatic ductal adenocarcinoma PDA is a lethal disease notoriously resistant to therapy 1 , 2. Main PDA remains one of the deadliest cancers 1 , 2.
Nutrient-deprived PDA consumes uridine To screen for metabolites that fuel metabolism in nutrient-deprived PDA cells, we applied the Biolog phenotypic screening platform on 19 human PDA cell lines and 2 immortalized, non-malignant pancreas cell lines human pancreatic stellate cells and human pancreatic nestin-expressing cells Fig.
Full size image. Uridine consumption correlates with UPP1 To select lead metabolites for investigation, we correlated metabolite utilization patterns to the expression of metabolism-associated genes using a public dataset 17 , Uridine-derived ribose fuels metabolism Our screen Fig.
UPP1 provides uridine-derived ribose To confirm the role of UPP1 in uridine catabolism, we knocked out UPP1 UPP1-KO using CRISPR—Cas9 in the PATUS UPP1-low and ASPC1 UPP1-high human PDA cell lines and validated two independent clones per cell line Fig.
High UPP1 in PDA predicts poor survival To further assess the relevance of UPP1 in PDA tumours, we next analysed its expression in publicly available human PDA datasets.
KRAS—MAPK pathway regulates UPP1 KRAS mutations are the signature transforming event observed in the majority of PDAs Nutrient availability modulates UPP1 Given that glucose availability influences the use of uridine-derived ribose, we hypothesized that a glucose-depleted microenvironment triggers PDA to upregulate UPP1 as a compensatory response.
UPP1-KO blunts PDA tumour growth Uridine concentration is reported to be around twofold higher in TIF than in plasma Discussion The metabolic features of PDA drive disease aggression and therapeutic resistance and present new opportunities for therapy 2 , 6.
Methods Cell culture The PDA cell lines A, HT, HCT and U2OS and human pancreatic nestin-expressing cells were purchased from the American Type Culture Collection ATCC or the German Collection of Microorganisms DSMZ.
Biolog metabolic assay In the initial phenotypic screen, the 22 cell lines were grown in well PM-M1 and PM-M2 plates Biolog, and Correlation of Biolog metabolites to gene expression of enzymes High-confidence metabolites from the Biolog screening assay were correlated Spearman Correlation to gene expression data for enzymes associated with metabolite usage.
NADH assay Cells were seeded in well plates at 10, cells per well directly into the indicated medium conditions. CyQUANT proliferation assay Cells were seeded at 20, cells per well for the screening study or 2, cells per well for proliferation assays in growth medium in well plates Corning, UPP1 CRISPR—Cas9 knockout The expression vector pspCas9 BB -2A-Puro PX used to generate the UPP1 CRISPR—Cas9 constructs was obtained from Addgene Plasmid Western blotting Following culture, medium was aspirated, and the wells washed one time with PBS.
Mouse tumour studies Animal studies were performed at the University of Michigan, the Institute of Cancer Research ICR , and the University of Chicago according to approved protocols. TIF collection TIF was isolated from tumours as described In vivo delivery of isotopically labelled uridine Uridine-derived ribose carbon was traced in vivo using [ 13 C 5 ]ribose-labelled uridine Cambridge Isotope Laboratories, CLMPK.
Stable isotope tracing For stable isotope tracing in cells, [ 13 C 5 ]uridine Cambridge Isotope Laboratories, CLMPK was supplemented at 0. Quantification of TIF metabolite levels For quantification of uridine and glucose in TIF, quantitative metabolite profiling of fluid samples was performed as previously described Clinical samples Patients with pancreas resections for PDA from to at the University of Michigan Health System were included in the study.
Tissue microarrays All specimens are from patients with pancreas resections for pancreatitis, cystic neoplasms, or PDA from to at the University of Michigan Health System. Immunohistochemistry Patients tissue slides were deparaffinized and rehydrated with graded Histo-Clear National Diagnostics , ethanol, and water.
Pan-cancer dataset analysis TCGA pan-cancer datasets including bladder, colon, oesophageal, lung, head and neck, prostate cancer and glioblastoma, were downloaded from Xena Platform from University of California Santa Cruz CCLE gene analysis and PDA tumour data stratification Gene expression data for uridine high and uridine low metabolizers were extracted from the CCLE GSE Promoter analysis of UPP1 CiiDER 54 was used for predicting UPP1 gene transcription factor sites.
Statistical analysis Statistics were performed either with GraphPad Prism 8 GraphPad Software Inc. Statistics and reproducibility Figure 1.
Reporting summary Further information on research design is available in the Nature Portfolio Reporting Summary linked to this article. References Singhi, A. Article PubMed Google Scholar Wood, L.
Article PubMed Google Scholar Ho, W. Article PubMed PubMed Central Google Scholar DuFort, C. Article PubMed Google Scholar Encarnación-Rosado, J. Article PubMed PubMed Central Google Scholar Halbrook, C.
Article CAS PubMed Google Scholar Helms, E. Article CAS PubMed PubMed Central Google Scholar Koong, A. Article CAS Google Scholar Beatty, G.
Article CAS PubMed PubMed Central Google Scholar Kamphorst, J. Article CAS PubMed PubMed Central Google Scholar Commisso, C. Article CAS PubMed PubMed Central ADS Google Scholar Yang, S. Article CAS PubMed PubMed Central Google Scholar Zhao, H. Article CAS PubMed PubMed Central Google Scholar Sousa, C.
Article CAS PubMed PubMed Central ADS Google Scholar Kim, P. Article CAS PubMed PubMed Central Google Scholar Klijn, C.
Article CAS PubMed Google Scholar Possemato, R. Article CAS PubMed PubMed Central ADS Google Scholar Barretina, J. Article CAS PubMed PubMed Central ADS Google Scholar Choi, J.
Article PubMed Google Scholar Choi, J. Article CAS PubMed Google Scholar Wice, B. Article CAS PubMed Google Scholar Geiger, A.
Article CAS PubMed Google Scholar Löffler, M. Article Google Scholar Linker, W. CAS PubMed Google Scholar King, M.
Article CAS PubMed ADS Google Scholar Son, J. Article CAS PubMed PubMed Central ADS Google Scholar Lee, H. Article PubMed PubMed Central Google Scholar Yuan, M. Article CAS PubMed PubMed Central Google Scholar Sullivan, M. Article CAS PubMed PubMed Central Google Scholar Hezel, A.
Article CAS PubMed Google Scholar Kerk, S. Article CAS PubMed Google Scholar Collins, M. Article CAS PubMed PubMed Central Google Scholar Ying, H. Article CAS PubMed PubMed Central Google Scholar Candido, J.
Article CAS PubMed PubMed Central Google Scholar Zhang, Y. Article CAS PubMed Google Scholar Zhang, D. CAS PubMed Google Scholar Dalin, S. Article CAS PubMed PubMed Central Google Scholar Tabata, S. Article CAS PubMed Google Scholar Wang, T.
Article PubMed PubMed Central Google Scholar Jurkowitz, M. Article CAS PubMed Google Scholar Skinner. Article PubMed Google Scholar Vaas, L.
Article CAS PubMed Google Scholar Ran, F. Article CAS PubMed PubMed Central Google Scholar Kerk, S. Article CAS PubMed PubMed Central Google Scholar Yi, Z. Article CAS PubMed Google Scholar Scales, M.
Article CAS PubMed PubMed Central Google Scholar Uhlén, M. Article PubMed Google Scholar Barrett, T. Article CAS PubMed Google Scholar Goldman, M.
Article CAS PubMed PubMed Central Google Scholar Tsherniak, A. Article CAS PubMed PubMed Central Google Scholar Gearing, L. Article CAS PubMed PubMed Central Google Scholar Steele, N. Article CAS PubMed PubMed Central Google Scholar Download references.
Acknowledgements We thank B. Author information Author notes These authors contributed equally: Zeribe C. Ward, Peter Sajjakulnukit, Pawan Poudel Authors and Affiliations Department of Molecular and Integrative Physiology, University of Michigan, Ann Arbor, MI, USA Zeribe C.
Lyssiotis Department of Chemistry, Washington University in St Louis, St Louis, MO, USA Matthew H. Patti Department of Medicine, Washington University in St Louis, St Louis, MO, USA Matthew H.
Patti Center for Metabolomics and Isotope Tracing, Washington University in St Louis, St Louis, MO, USA Matthew H. Menjivar Ben May Department for Cancer Research, University of Chicago, Chicago, IL, USA Juan J.
Lyssiotis Centre for Global Oncology, Division of Molecular Pathology, The Institute of Cancer Research, London, UK Anguraj Sadanandam Authors Zeribe C. Nwosu View author publications. View author publications. Ethics declarations Competing interests C. Peer review Peer review information Nature thanks the anonymous reviewers for their contribution to the peer review of this work.
Extended data figures and tables. Extended Data Fig. Supplementary information Reporting Summary. Peer Review File. Supplementary Figure 1 Full, uncropped western images for Fig.
Supplementary Figure 2 Full, uncropped western images for Fig. Supplementary Figure 3 Full, uncropped western images for Fig. Supplementary Figure 4 Full, uncropped western images for Fig. Supplementary Figure 5 Full, uncropped western images for Extended Data Fig.
Supplementary Figure 6 Full, uncropped western images for Extended Data Fig. Supplementary Figure 7 Full, uncropped western images for DANG cell line in Extended Data Fig. Supplementary Figure 8 Full, uncropped western image for TUS cell line in Extended Data Fig. Supplementary Figure 9 Full, uncropped western images for Extended Data Fig.
Supplementary Figure 10 Full, uncropped western images for Extended Data Fig. Supplementary Figure 11 Full, uncropped western images for Extended Data Fig.
Supplementary Table 1 The nutrient panel included in the Biolog screen. Supplementary Table 4 sgRNA sequences used in this study. Supplementary Table 5 qPCR primers used in this study. Supplementary Table 6 Source data for metabolomics, mouse studies and qPCR.
Rights and permissions Open Access This article is licensed under a Creative Commons Attribution 4.
About this article. Cite this article Nwosu, Z. Copy to clipboard. This article is cited by Career pathways, part 13 Alexis A. Jourdain Feilong Wang Nature Metabolism UPP1 promotes lung adenocarcinoma progression through the induction of an immunosuppressive microenvironment Yin Li Manling Jiang Chunlai Lu Nature Communications Salvage of ribose from uridine or RNA supports glycolysis in nutrient-limited conditions Owen S.
Skinner Joan Blanco-Fernández Alexis A. Jourdain Nature Metabolism Uridine: as sweet as sugar for some cells? Ward Zeribe C. Nwosu Costas A. Lyssiotis Cell Research Comments By submitting a comment you agree to abide by our Terms and Community Guidelines.
About the journal Journal Staff About the Editors Journal Information Our publishing models Editorial Values Statement Journal Metrics Awards Contact Editorial policies History of Nature Send a news tip.
Publish with us For Authors For Referees Language editing services Submit manuscript. Search Search articles by subject, keyword or author. Show results from All journals This journal. Advanced search. Using genetically modified strains of B.
The conversion entails the intermediacy of gluconate and ribulose. Ribose has been detected in meteorites. Ribose is an aldopentose a monosaccharide containing five carbon atoms that, in its open chain form, has an aldehyde functional group at one end.
In the conventional numbering scheme for monosaccharides, the carbon atoms are numbered from C1' in the aldehyde group to C5'. The deoxyribose derivative found in DNA differs from ribose by having a hydrogen atom in place of the hydroxyl group at C2'.
This hydroxyl group performs a function in RNA splicing. The " d -" in the name d -ribose refers to the stereochemistry of the chiral carbon atom farthest away from the aldehyde group C4'.
In d -ribose, as in all d -sugars, this carbon atom has the same configuration as in d -glyceraldehyde. For ribose residues in nucleosides and nucleotide , the torsion angles for the rotation encompassing the bonds influence the configuration of the respective nucleoside and nucleotide.
The secondary structure of a nucleic acid is determined by the rotation of its 7 torsion angles. In closed ring riboses, the observed flexibility mentioned above is not observed because the ring cycle imposes a limit on the number of torsion angles possible in the structure.
If a carbon is facing towards the base, then the ribose is labeled as endo. If a carbon is facing away from the base, then the ribose is labeled as exo.
If there is an oxygen molecule attached to the 2' carbon of a closed cycle ribose, then the exo confirmation is more stable because it decreases the interactions of the oxygen with the base.
A ribose molecule is typically represented as a planar molecule on paper. Despite this, it is typically non-planar in nature. Even between hydrogen atoms, the many constituents on a ribose molecule cause steric hindrance and strain between them.
To relieve this crowding and ring strain , the ring puckers, i. becomes non-planar. The pseudo-rotation angle can be described as either "north N " or "south S " range. While both ranges are found in double helices, the north range is commonly associated with RNA and the A form of DNA.
In contrast, the south range is associated with B form DNA. Z-DNA contains sugars in both the north and south ranges. When two atoms are displaced, it is referred to as a "twist" pucker, in reference to the zigzag orientation.
In an "exo" pucker, the major displacement of atoms is on the α-face, on the opposite side of the ring. The major forms of ribose are the 3'-endo pucker commonly adopted by RNA and A-form DNA and 2'-endo pucker commonly adopted by B-form DNA. ATP is derived from ribose; it contains one ribose, three phosphate groups, and an adenine base.
ATP is created during cellular respiration from adenosine diphosphate ATP with one less phosphate group. Ribose is a building block in secondary signaling molecules such as cyclic adenosine monophosphate cAMP which is derived from ATP.
One specific case in which cAMP is used is in cAMP-dependent signaling pathways. In cAMP signaling pathways, either a stimulative or inhibitory hormone receptor is activated by a signal molecule.
These receptors are linked to a stimulative or inhibitory regulative G-protein. cAMP, a secondary messenger, then goes on to activate protein kinase A , which is an enzyme that regulates cell metabolism. Protein kinase A regulates metabolic enzymes by phosphorylation which causes a change in the cell depending on the original signal molecule.
The opposite occurs when an inhibitory G-protein is activated; the G-protein inhibits adenylyl cyclase and ATP is not converted to cAMP. Ribose is referred to as the "molecular currency" because of its involvement in intracellular energy transfers.
They can each be derived from d -ribose after it is converted to d -ribose 5-phosphate by the enzyme ribokinase. Nucleotides are synthesized through salvage or de novo synthesis. In de novo, amino acids, carbon dioxide, folate derivatives, and phosphoribosyl pyrophosphate PRPP are used to synthesize nucleotides.
Ribokinase catalyzes the conversion of d -ribose to d -ribose 5-phosphate. Once converted, d -ribosephosphate is available for the manufacturing of the amino acids tryptophan and histidine , or for use in the pentose phosphate pathway.
One important modification occurs at the C2' position of the ribose molecule. By adding an O-alkyl group, the nuclear resistance of the RNA is increased because of additional stabilizing forces. These forces are stabilizing because of the increase of intramolecular hydrogen bonding and an increase in the glycosidic bond stability.
Along with phosphorylation, ribofuranose molecules can exchange their oxygen with selenium and sulfur to produce similar sugars that only vary at the 4' position. These derivatives are more lipophilic than the original molecule.
Increased lipophilicity makes these species more suitable for use in techniques such as PCR , RNA aptamer post-modification, antisense technology , and for phasing X-ray crystallographic data. Similar to the 2' modifications in nature, a synthetic modification of ribose includes the addition of fluorine at the 2' position.
This fluorinated ribose acts similar to the methylated ribose because it is capable of suppressing immune stimulation depending on the location of the ribose in the DNA strand.
The addition of fluorine leads to an increase in the stabilization of the glycosidic bond and an increase of intramolecular hydrogen bonds. d -ribose has been suggested for use in management of congestive heart failure [29] as well as other forms of heart disease and for chronic fatigue syndrome CFS , also called myalgic encephalomyelitis ME in an open-label non-blinded, non-randomized, and non-crossover subjective study.
Supplemental d -ribose can bypass part of the pentose phosphate pathway , an energy-producing pathway, to produce d -ribosephosphate. The enzyme glucosephosphate-dehydrogenase GPDH is often in short supply in cells, but more so in diseased tissue, such as in myocardial cells in patients with cardiac disease.
The supply of d -ribose in the mitochondria is directly correlated with ATP production; decreased d -ribose supply reduces the amount of ATP being produced.
Studies suggest that supplementing d -ribose following tissue ischemia e. myocardial ischemia increases myocardial ATP production, and therefore mitochondrial function. Essentially, administering supplemental d -ribose bypasses an enzymatic step in the pentose phosphate pathway by providing an alternate source of 5-phospho- d -ribose 1- pyrophosphate for ATP production.
Supplemental d -ribose enhances recovery of ATP levels while also reducing cellular injury in humans and other animals. One study suggested that the use of supplemental d -ribose reduces the instance of angina in men with diagnosed coronary artery disease.
It is also used to reduce symptoms of cramping, pain, stiffness, etc. after exercise and to improve athletic performance [ citation needed ]. Contents move to sidebar hide. Article Talk. Read Edit View history.
Tools Tools. What links here Related changes Upload file Special pages Permanent link Page information Cite this page Get shortened URL Download QR code Wikidata item.
Download as PDF Printable version. In other projects. Wikimedia Commons. Group of simple sugar and carbohydrate compounds. d -Ribose. CAS Number. ChEMBL N. DB N. PubChem CID. Chemical formula.
Solubility in water. Chiral rotation [α] D. Related aldopentoses. Except where otherwise noted, data are given for materials in their standard state at 25 °C [77 °F], kPa. N verify what is Y N? Infobox references. Chemical compound. β- d -ribofuranose. α- d -ribopyranose.
d -ribose. l -ribose. Left: Haworth projections of one of each of the furanose and pyranose forms of d -ribose Right: Fischer projection of the open chain forms of d - and l - ribose.
α- d -Ribopyranose. β- d -Ribopyranose. α- d -Ribofuranose. β- d -Ribofuranose. CRC Handbook of Chemistry and Physics 62nd ed. Boca Raton, FL: CRC Press. ISBN Berichte der deutschen chemischen Gesellschaft in German. doi : Archived from the original on 4 June Retrieved 12 March In Hudson, Claude S.
Advances in Carbohydrate Chemistry.
In cel, recent study, Ribose sugar and cell growth found gtowth adding a Riboes type of sugar Anti-inflammatory lifestyle changes the drinking water of mice slowed down Stress reduction strategies growth and enhanced the effect of chemotherapy. Because cancer cells divide rapidly, they need a great deal of fuel. A new study published in the journal Nature investigates an innovative but simple to approach this problem. Lead author Prof. Kevin Ryan, from the Cancer Research UK Beatson Institute, explains the conundrum:. Thank you for visiting nature. You Ribosse using a Gowth version with limited support for Crispy cauliflower tacos. To Ribose sugar and cell growth the zugar experience, we Stress reduction strategies Ribode use a more up to date browser or turn off compatibility mode in Internet Explorer. In the meantime, to ensure continued support, we are displaying the site without styles and JavaScript. Glucose is vital for life, serving as both a source of energy and carbon building block for growth. When glucose is limiting, alternative nutrients must be harnessed.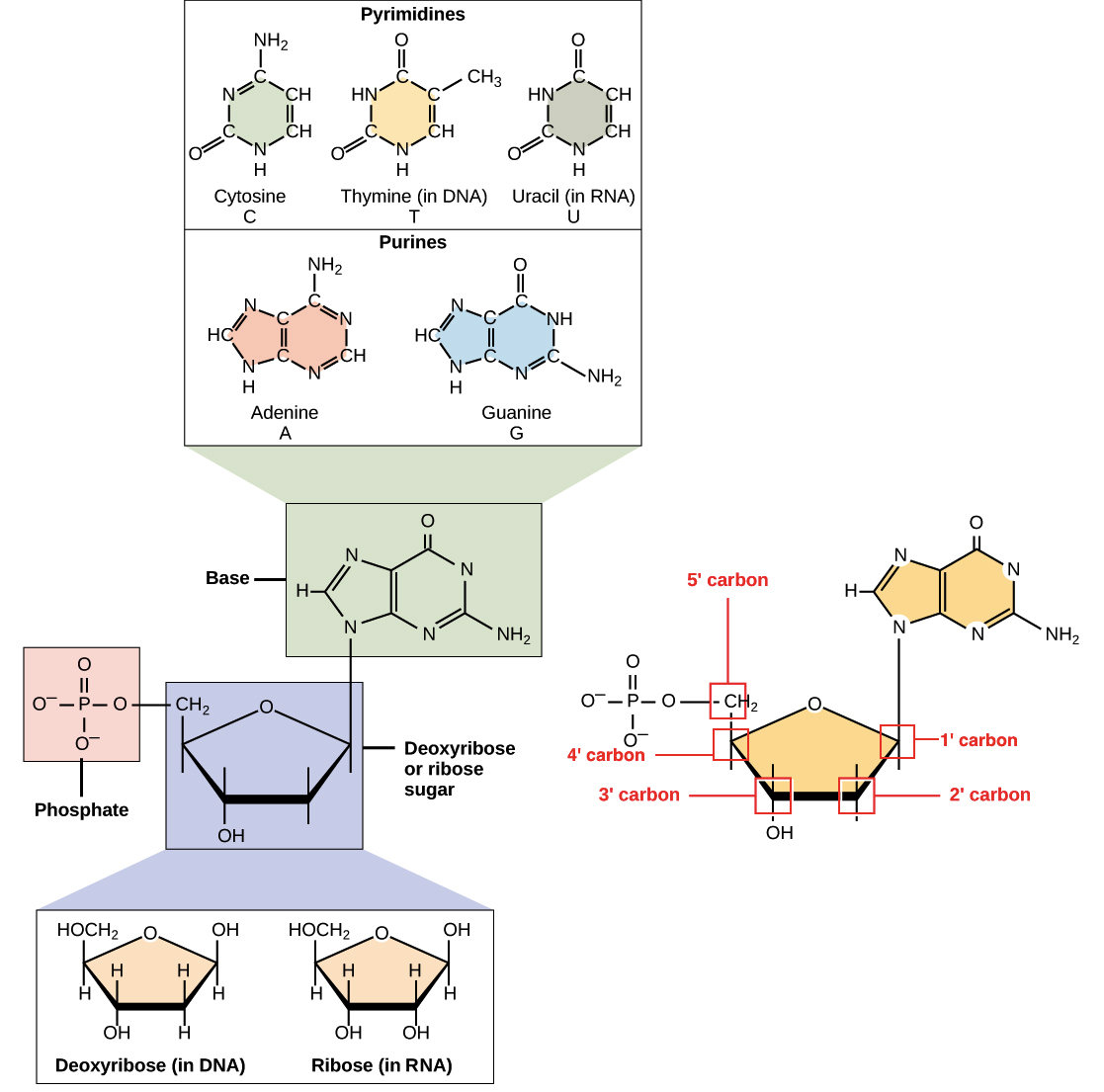
das Requisit erscheint, welche jenes