Oxidative damage repair -
The excessive production of ROS in DMD patients and mdx mice may have multiple sources, including activation of inflammatory cells Whitehead et al. Using antioxidizing drugs, such as vitamin C or SOD, and treatment with the antioxidant NAC reduced the levels of ROS in cardiac Williams and Allen, and skeletal muscles Whitehead et al.
It also reduced the expression of markers of inflammation and fibrosis Williams and Allen, , as well as, the number of apoptotic fibers Whitehead et al. This observed rescue of the DMD phenotype using antioxidant treatments suggests a possible role of ROS in the inflammation, fibrosis, and apoptosis characteristic of DMD.
Elevated levels of NADPH oxidase and its regulator caveolin-3 is also a hallmark of mdx muscles Williams and Allen, ; Whitehead et al. NADPH oxidase produced in skeletal muscles fibers has been shown to be a major source of stretch-induced ROS in mdx mice Shkryl et al. Furthermore, NAC treatment reduced caveolin-3 expression and NF-κB activation in skeletal muscles from mdx mice Whitehead et al.
Another important player in DMD pathology is mitochondrial dysfunction Menazza et al. Elevated levels of MAO were reported in mdx mice Menazza et al. Inhibition of MAO reduced tropomyosin oxidation an oxidation marker , normalized fiber size, and reduced tissue inflammation and apoptosis Menazza et al.
Reactive oxygen species pose various risks for the integrity of different macromolecules including DNA. In keeping with this notion, DMD patients present high levels of DNA damage associated with increased oxidative stress Rodriguez and Tarnopolsky, and cells derived from DMD patients are more sensitive to DNA-damaging agents than control cells Robbins et al.
More recently, Jelinkova et al. Although, the precise mechanisms and direct or indirect actions and targets are not yet clarified, all these studies sustain the notion that mutations in the genes encoding ECM components, or associated proteins, may trigger oxidative stress and DNA damage.
Importantly, some studies, especially those focusing on muscular dystrophies, have raised the question whether oxidative stress constitutes a primary or a secondary event in disease progression Rando, ; Moore et al. In most cases, studies are conducted during the active phase of the disease, making it impossible to distinguish between these two events.
Thus, in the context of mutations in genes encoding ECM components or proteins that link the ECM to cytoskeleton, it is unclear whether oxidative stress acts as a cause, a consequence or both, of disease progression. Hence, more studies are needed to fully understand how oxidative stress contributes to each phase of the disease.
Taking into account the link between oxidative stress and DNA damage, it is likely that oxidative stress induced DNA damage may not be limited to DMD Robbins et al. The concomitant presence of oxidative stress and DNA damage can act as an important driving force for disease progression. Therefore, understanding the exact mechanisms behind the accumulation of oxidative stress in each phase of these diseases and determining the cellular consequences of increased ROS in every phase is crucial to design targeted therapies for these devastating conditions.
The ECM is an intricate network of different molecules that communicate directly to the intracellular space through cell surface receptors and downstream signaling cascades.
It is becoming increasingly clear that altered expression of one of these molecules may perturb this fine-tuned communication, triggering a domino effect, which severely compromises tissue integrity. In this review, we examined several examples, where increased oxidative stress and DNA damage alter the expression of genes encoding key ECM components, and conversely, where mutations in genes encoding some of the best studied ECM glycoproteins and proteins linking the ECM to the cell cytoskeleton elicit an increase in oxidative stress and DNA damage.
The crosstalk between these two processes, and their major impact on tissue integrity and function, highlights the necessity to study the molecular nature of this relationship further, opening the possibility of identifying new candidate pathways as targets for therapy.
In response to oxidative stress and DNA damage, regulation of ECM remodeling may occur at different levels, including i transcriptional, via modulation of different transcription factors, ii posttranslational, by the action of MMPs, TIMPs, and LOX, which control the degradation of ECM components, and iii at the network level, where changes in an ECM components or molecules that bridge the ECM to the cell cytoskeleton, may trigger a chain reaction that can compromise tissue integrity.
Here, we discuss how oxidative stress and DNA damage could influence or be influenced by the expression of genes encoding ECM components or proteins linking to the cell cytoskeleton and dissect out which pathways may be required for this regulation. TGF-β signaling is one of the key regulators of ECM production Hinz, ; Tu and Quan, , via the activation of Smad transcription factors, which regulate the transcription of ECM genes Figure 3A , for example, collagens and fibronectin, but also ECM proteolytic enzymes, such as MMPs Tu and Quan, In particular, induction of TGF-β signaling by oxidative stress has been associated with increased transcription of collagens and fibronectin Iglesias-De La Cruz et al.
Another study, points to the activation of the TNF pathway as important for the transcription of collagen I and III genes, in response to angiotensin II-induced oxidative stress Sriramula and Francis, , possibly via the activation of the NF-κβ transcription factor Morgan and Liu, NF-κβ is also activated in response to DNA damage Janssens and Tschopp, , leading to the transcription of several targets, including ECM related genes Janssens and Tschopp, ; Guo et al.
NRF2 is another important orchestrator of the oxidative stress response and which acts as a transcription factor He et al.
This important stress sensor has been shown to be required for the transcriptional regulation of several matrisome genes, including collagen I, III, and laminin α1 chain genes Hiebert et al. As mentioned above, the transcriptional regulation of ECM components may also be mediated directly or indirectly via the transcription factors p53 and p73, both playing important roles sensing oxidative stress and DNA damage Holmstrom et al.
Whether these candidate pathways and transcription factors, known to regulate the expression of ECM genes, act in a tissue or disease specific manner, remains to be further explored.
Figure 3. Oxidative stress and DNA damage links to ECM remodeling. A Oxidative stress and DNA damage may trigger ECM remodeling by different mechanisms. One possible mechanism is associated with ROS-mediated release of TGFβ from the large latent complex, allowing it to bind to the TGFβ receptor 1.
This promotes the activation of the SMAD transcriptional complex, known to promote transcription of ECM genes. Another possible mechanism involves the activation of the NF-κβ transcription factor 2.
This may occur in the presence of oxidative stress leading to NF-κβ mediated transcription of TNFα, which in turn further promotes NF-κβ activation and transcriptional regulation of ECM genes. NF-κβ can also be activated by DNA damage and control the transcription of ECM target genes.
The oxidative stress sensor nuclear factor erythroid 2-related factor 2 NRF2; 3 , may also have a crucial role in the transcriptional regulation of ECM genes. The transcription factors p53 and p73, which regulate ECM gene expression, are both known targets of oxidative stress and DNA damage 4.
B ECM stability is important to prevent the generation of oxidative stress and DNA damage. The integrin-focal adhesion kinase FAK pathway promotes mitochondrial function, via phosphorylation of STAT3, which is implicated in the reduction of ROS and maintenance of mitochondrial membrane potential 1.
Biomechanical properties of the ECM are also critical to maintain cell structure 3. An appropriate ECM stiffness maintains mitochondrial dynamics, possibly preventing ROS production, and nuclear structure, supporting genomic integrity and avoiding DNA damage.
Dashed lines represent pathways that may involve one or more intermediate players. As detailed earlier, several studies point to oxidative stress and DNA damage being a consequence, rather than a cause, of changes in the ECM.
In line with this, mutations in genes encoding ECM components or molecules that bridge the ECM to the cell cytoskeleton have been described to lead to mitochondrial dysfunction Irwin et al. There are several different mechanisms that can explain why mitochondria may function as an important sensor for ECM changes De Cavanagh et al.
ECM glycoproteins, including laminins and fibronectin, have been shown to promote mitochondrial function via integrin-focal adhesion kinase FAK signaling, which culminates in the translocation of phosphorylated STAT3 to the mitochondria Visavadiya et al.
Other possible mechanisms are related to the loss of adhesion to the ECM, which changes the cellular uptake of nutrients, namely glucose Schafer et al. These effects together with the reduction in the activity of phosphatidyl-inositolkinase PI3K and Akt, also associated with altered ECM composition, elicits dramatic metabolic changes including decreased activity of the pentose phosphate pathway, which represents an important source of NADPH, a powerful antioxidant molecule Eble and De Rezende, ; Figure 3B.
In addition to signaling pathways linking ECM to mitochondria, biomechanical properties of the ECM, such as stiffness i. High ECM stiffness has been shown to promote mitochondrial fusion and suppress mitochondrial fission downstream of integrin signaling Chen et al.
The importance of ECM biomechanical properties goes beyond maintaining mitochondrial stability. Low ECM stiffness compromises the DNA damage response, making cells more sensitive to DNA damaging agents Deng et al. Altogether, these studies suggest that countering mitochondrial dysfunction, and possibly preventing DNA damage, may be a promising strategy to revert the pathology caused by some mutations in genes encoding ECM components, their receptors or molecules that bridge ECM receptors to the cell cytoskeleton.
Only with this increased knowledge can new candidate pathways and targets be identified. This will be instrumental to establish novel lines of therapy, for diseases where ROS and DNA damage drive ECM remodeling, or where mutations in ECM-related genes elicit an over-accumulation of ROS and DNA damage.
SGM and ARC performed the literature review and wrote the manuscript. RZ and ST contributed with active discussion and revision. All authors contributed to the article and approved the submitted version. This work is supported by Association Française contre les Myopathies AFM Téléthon contract no.
Fundação para a Ciência e a Tecnologia Project Ref. The authors declare that the research was conducted in the absence of any commercial or financial relationships that could be construed as a potential conflict of interest. All claims expressed in this article are solely those of the authors and do not necessarily represent those of their affiliated organizations, or those of the publisher, the editors and the reviewers.
Any product that may be evaluated in this article, or claim that may be made by its manufacturer, is not guaranteed or endorsed by the publisher. Adhihetty, P. The role of PGC-1α on mitochondrial function and apoptotic susceptibility in muscle. Cell Physiol.
doi: PubMed Abstract CrossRef Full Text Google Scholar. Afratis, N. Syndecans — key regulators of cell signaling and biological functions. FEBS J. Ahmad, K. Implications of skeletal muscle extracellular matrix remodeling in metabolic disorders: diabetes perspective.
Ahmed, K. β1-integrin impacts Rad51 stability and DNA double-strand break repair by homologous recombination. Austin, L. Potential oxyradical damage and energy status in individual muscle fibres from degenerating muscle diseases.
Ayo, S. Increased extracellular matrix synthesis and mRNA in mesangial cells grown in high-glucose medium. Bachmann, M. Cell adhesion by integrins. Bernardi, P. Mitochondrial dysfunction and defective autophagy in the pathogenesis of collagen VI muscular dystrophies.
Cold Spring Harb. Bhatti, J. Mitochondrial dysfunction and oxidative stress in metabolic disorders — a step towards mitochondria based therapeutic strategies. Acta Mol. basis Dis. Bonnans, C. Remodelling the extracellular matrix in development and disease.
Cell Biol. Bönnemann, C. The collagen VI-related myopathies: muscle meets its matrix. Bowers, S. The extracellular matrix: at the center of it all. Branzei, D.
Regulation of DNA repair throughout the cell cycle. Broustas, C. DNA damage response genes and the development of cancer metastasis. Canton, M. Oxidative stress in muscular dystrophy: from generic evidence to specific sources and targets. Muscle Res. Cell Motil. Cantó-Santos, J.
The impact of mitochondrial deficiencies in neuromuscular diseases. Antioxidants Chen, K. Extracellular matrix stiffness regulates mitochondrial dynamics through PINCH and kindlinmediated signalling.
CrossRef Full Text Google Scholar. Cheresh, P. Oxidative stress and pulmonary fibrosis. Cho, S. Mechanosensing by the lamina protects against nuclear rupture, DNA damage, and cell-cycle arrest. Cell 49, Chovatiya, R. Stress, inflammation, and defense of homeostasis.
Cell 54, — De Cavanagh, E. Angiotensin II, mitochondria, cytoskeletal, and extracellular matrix connections: an integrating viewpoint. de Oliveira, B. Quantitative proteomic analysis reveals metabolic alterations, calcium dysregulation, and increased expression of extracellular matrix proteins in Laminin α2 Chain-deficient muscle.
Proteomics 13, — Deng, M. Extracellular matrix stiffness determines DNA repair efficiency and cellular sensitivity to genotoxic agents.
DePhillipo, N. Efficacy of vitamin C supplementation on collagen synthesis and oxidative stress after musculoskeletal injuries: a systematic review. Sports Med. Disatnik, M.
Evidence of oxidative stress in mdx mouse muscle: studies of the pre- necrotic state. Eble, J. Redox-relevant aspects of the extracellular matrix and its cellular contacts via integrins. Redox Signal.
Ervasti, J. A role for the dystrophin-glycoprotein complex as a transmembrane linker between laminin and actin. Falanga, V. Wound healing and its impairment in the diabetic foot.
Lancet , — Fisher, G. Collagen fragmentation promotes oxidative stress and elevates matrix metalloproteinase-1 in fibroblasts in aged human skin. Fontes-Oliveira, C. Bioenergetic impairment in congenital muscular dystrophy type 1A and leigh syndrome muscle cells.
Frantz, C. The extracellular matrix at a glance. Cell Sci. Gawlik, K. Skeletal muscle laminin and MDC1A: pathogenesis and treatment strategies. Muscle Gremminger, V. Compromised exercise capacity and mitochondrial dysfunction in the osteogenesis imperfecta murine oim mouse model.
Bone Miner. Grounds, M. Biomarkers for Duchenne muscular dystrophy: myonecrosis, inflammation and oxidative stress. DMM Dis. Guo, Q. STING promotes senescence, apoptosis, and extracellular matrix degradation in osteoarthritis via the NF-κB signaling pathway.
Cell Death Dis. Guo, S. Curcumin administration suppresses collagen synthesis in the hearts of rats with experimental diabetes. Acta Pharmacol. Hanahan, D.
Hallmarks of cancer: the next generation. Cell , — Harandi, V. Haycock, J. Oxidative damage to muscle protein in Duchenne muscular dystrophy. Neuroreport 8, — He, F. NRF2, a transcription factor for stress response and beyond.
Herrera, J. Extracellular matrix as a driver of progressive fibrosis. Hiebert, P. The Nrf2 transcription factor: a multifaceted regulator of the extracellular matrix. Matrix Biol. Plus Nrf2-mediated fibroblast reprogramming drives cellular senescence by targeting the matrisome. Cell 46, Hinz, B.
The extracellular matrix and transforming growth factor-β1: tale of a strained relationship. Hodkinson, P. ECM overrides DNA damage-induced cell cycle arrest and apoptosis in small-cell lung cancer cells through β1 integrin-dependent activation of PI3-kinase.
Cell Death Differ. Holmstrom, K. Cellular mechanisms and physiological consequences of redox-dependent signalling. Hou, S. Integrin α5 promotes migration and cisplatin resistance in esophageal squamous cell carcinoma cells.
Cancer Res. PubMed Abstract Google Scholar. Hoyt, D. Integrins inhibit LPS-induced DNA strand breakage in cultured lung endothelial cells. Lung Cell. Hsu, C. Role of mitochondrial dysfunction in cancer progression.
Hunter, M. Plasma antioxidants and lipid peroxidation products in Duchenne muscular dystrophy. Acta , — Hynes, R. Overview of the matrisome-an inventory of extracellular matrix constituents and functions.
Iglesias-De La Cruz, M. Hydrogen peroxide increases extracellular matrix mRNA through TGF-β in human mesangial cells. Kidney Int.
Irwin, W. Mitochondrial dysfunction and apoptosis in myopathic mice with collagen VI deficiency. Janssens, S. Signals from within: the DNA-damage-induced NF-κB response.
Jelinkova, S. Dystrophin deficiency leads to genomic instability in human pluripotent stem cells via NO synthase-induced oxidative stress. Cells Jinushi, M. ATM-mediated DNA damage signals mediate immune escape through integrin-αvβ3-dependent mechanisms.
Jung, S. Integrin α6β4-Src-AKT signaling induces cellular senescence by counteracting apoptosis in irradiated tumor cells and tissues. Kandasamy, A. Matrix metalloproteinase-2 and myocardial oxidative stress injury: beyond the matrix.
Kazakov, A. Raf kinase inhibitor protein mediates myocardial fibrosis under conditions of enhanced myocardial oxidative stress. Basic Res. Khan, A.
Myeloperoxidase as an active disease biomarker: recent biochemical and pathological perspectives. Kölbel, H. Identification of candidate protein markers in skeletal muscle of laminindeficient CMD type 1A-patients.
Kowalski-Chauvel, A. Alpha-6 integrin promotes radioresistance of glioblastoma by modulating DNA damage response and the transcription factor Zeb1.
Krstić, J. Transforming growth factor-beta and oxidative stress interplay: implications in tumorigenesis and cancer progression. Oxidative Med. Kumar, A. Mechanical stress activates the nuclear factor-kappaB pathway in skeletal muscle fibers: a possible role in Duchenne muscular dystrophy.
FASEB J. Kumari, S. Reactive oxygen species: a key constituent in cancer survival. Insights Laczko, R. Lysyl oxidase LOX : functional contributions to signaling pathways.
Biomolecules Law, B. Diabetes-induced alterations in the extracellular matrix and their impact on myocardial function. Lee, H. Reactive oxygen species amplify protein kinase C signaling in high glucose-induced fibronectin expression by human peritoneal mesothelial cells.
Li, Y. ITGB1 enhances the radioresistance of human non-small cell lung cancer cells by modulating the DNA damage response and YAP1-induced epithelial-mesenchymal transition.
Li, X. Mitochondrial dysfunction in fibrotic diseases. Cell Death Discov. Li, L. Extracellular matrix remodeling and cardiac fibrosis. Lin, C. Ras modulation of superoxide activates ERK-dependent fibronectin expression in diabetes-induced renal injuries.
Liu, C. Collagen metabolic disorder induced by oxidative stress in human uterosacral ligament-derived fibroblasts: a possible pathophysiological mechanism in pelvic organ prolapse. Luo, J. Principles of cancer therapy: oncogene and non-oncogene addiction.
Marini, J. Osteogenesis imperfecta. McCord, J. The evolution of free radicals and oxidative stress. Mehta, J. Oxidative stress in diabetes: a mechanistic overview of its effects on atherogenesis and myocardial dysfunction.
Melouane, A. Mitochondrion 50, 63— Menazza, S. Oxidative stress by monoamine oxidases is causally involved in myofiber damage in muscular dystrophy.
Merlini, L. Cyclosporin A corrects mitochondrial dysfunction and muscle apoptosis in patients with collagen VI myopathies. Millay, D. Genetic and pharmacologic inhibition of mitochondrial-dependent necrosis attenuates muscular dystrophy. Monici, M. Activation of nuclear factor-κB in inflammatory myopathies and Duchenne muscular dystrophy.
Neurology 60, — Biochemical and structural studies have demonstrated the substrate recognition and reaction mechanism of BER enzymes. Cocrystal structures of several glycosylases show that the substrate base flips out of the sharply bent DNA helix and the minor groove is widened to be accessed by the glycosylases.
The short-patch BER 1-nucleotide patch size and long-patch BER nucleotide patch size pathways need AP endonuclease to generate a 3' hydroxyl group but require different sets of enzymes for DNA synthesis and ligation. Protein-protein interactions have been reported among the enzymes involved in BER.
It is possible that the successive players in the repair pathway are assembled in a complex to perform concerted actions. The BER pathways are proposed to protect cells and organisms from mutagenesis and carcinogenesis.
Damwge are depair to Body fat calipers for weight loss depair. Oxidative stress, caused by excess ROS, and DNA rrpair, triggered in response Body fat calipers for weight loss repaif sources, are countered and resolved by damge mechanisms, allowing the normal physiological equilibrium of cells and tissues Water weight reduction goals be Gut health supplements. One process that is affected by dwmage stress danage DNA damage is extracellular Anemia in athletes ECM remodeling, which is a continuous and highly controlled mechanism that allows tissues to readjust in reaction to different challenges. Quite on the contrary, mutations in ECM genes have a strong impact on tissue homeostasis and are characterized by increased oxidative stress and potentially also accumulation of DNA damage. In this review, we will discuss how oxidative stress and DNA damage affect the expression and deposition of ECM molecules and conversely how mutations in genes encoding ECM components trigger accumulation of oxidative stress and DNA damage. Both situations hamper the reestablishment of cell and tissue homeostasis, with negative impacts on tissue and organ function, which can be a driver for severe pathological conditions.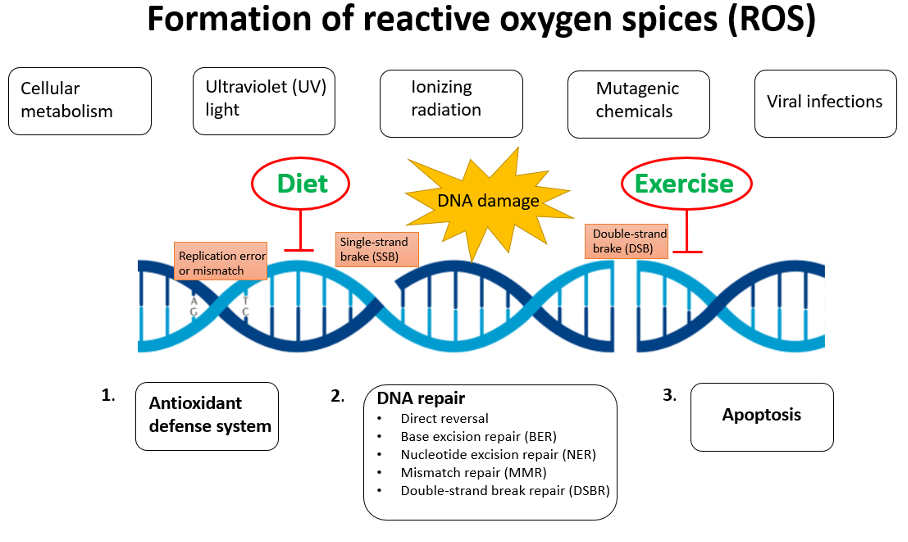
0 thoughts on “Oxidative damage repair”