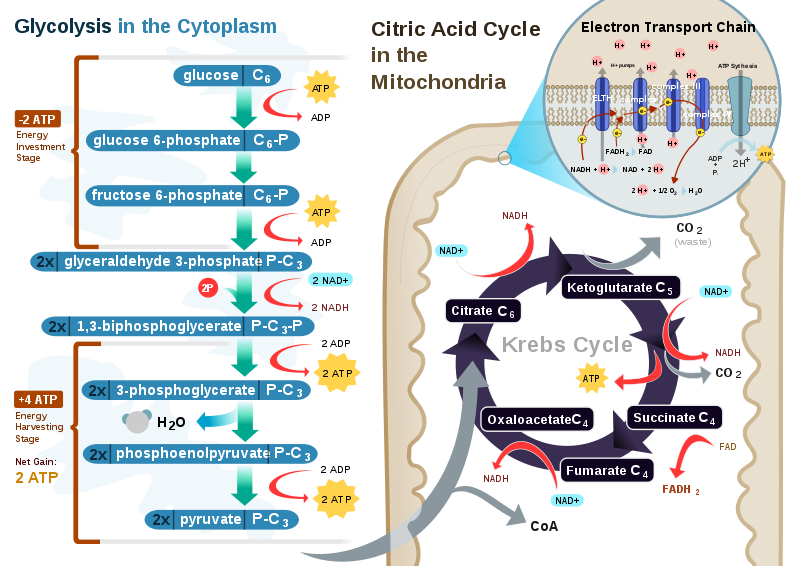
Energy metabolism and macronutrients -
To ensure cellular efficiency, the metabolic pathways involved in catabolism and anabolism are regulated in concert by energy status, hormones, and substrate and end-product levels. The concerted regulation of metabolic pathways prevents cells from inefficiently building a molecule when it is already available.
Just as it would be inefficient to build a wall at the same time as it is being broken down, it is not metabolically efficient for a cell to synthesize fatty acids and break them down at the same time.
Catabolism of food molecules begins when food enters the mouth, as the enzyme salivary amylase initiates the breakdown of the starch in foods. The entire process of digestion converts the large polymers in food to monomers that can be absorbed.
Starches are broken down to monosaccharides, lipids are broken down to fatty acids, and proteins are broken down to amino acids. These monomers are absorbed into the bloodstream either directly, as is the case with monosaccharides and amino acids, or repackaged in intestinal cells for transport by an indirect route through lymphatic vessels, as is the case with most fatty acids and other fat-soluble molecules.
Once absorbed, water-soluble nutrients first travel to the liver which controls their passage into the blood that transports the nutrients to cells throughout the body. The fat-soluble nutrients gradually pass from the lymphatic vessels into blood flowing to body cells.
Cells requiring energy or building blocks take up the nutrients from the blood and process them in either catabolic or anabolic pathways.
The organ systems of the body require fuel and building blocks to perform the many functions of the body, such as digesting, absorbing, breathing, pumping blood, transporting nutrients in and wastes out, maintaining body temperature, and making new cells.
Energy metabolism refers more specifically to the metabolic pathways that release or store energy. Some of these are catabolic pathways, like glycolysis the splitting of glucose , β-oxidation fatty-acid breakdown , and amino acid catabolism. Others are anabolic pathways, and include those involved in storing excess energy such as glycogenesis , and synthesizing triglycerides lipogenesis.
Table 3. All cells are in tune to their energy balance. When energy levels are high cells build molecules, and when energy levels are low catabolic pathways are initiated to make energy. Glucose is the preferred energy source by most tissues, but fatty acids and amino acids also can be catabolized to release energy that can drive the formation of ATP.
ATP is a high energy molecule that can drive chemical reactions that require energy. The catabolism of nutrients to release energy can be separated into three stages, each containing individual metabolic pathways. The three stages of nutrient breakdown are the following:.
The breakdown of glucose begins with glycolysis, which is a ten-step metabolic pathway yielding two ATP per glucose molecule; glycolysis takes place in the cytosol and does not require oxygen.
In addition to ATP, the end-products of glycolysis include two three-carbon molecules, called pyruvate. Pyruvate can either be shuttled to the citric acid cycle to make more ATP or follow an anabolic pathway. If a cell is in negative-energy balance, pyruvate is transported to the mitochondria where it first gets one of its carbons chopped off, yielding acetyl-CoA.
The breakdown of fatty acids begins with the catabolic pathway, known as β-oxidation, which takes place in the mitochondria. In this catabolic pathway, four enzymatic steps sequentially remove two-carbon molecules from long chains of fatty acids, yielding acetyl-CoA molecules.
In the case of amino acids, once the nitrogen is removed from the amino acid the remaining carbon skeleton can be enzymatically converted into acetyl-CoA or some other intermediate of the citric acid cycle.
Acetyl-CoA, a two-carbon molecule common to glucose, lipid, and protein metabolism enters the second stage of energy metabolism, the citric acid cycle. In the citric acid cycle, acetyl-CoA is joined to a four-carbon molecule. In this multistep pathway, two carbons are lost as two molecules of carbon dioxide.
The energy obtained from the breaking of chemical bonds in the citric acid cycle is transformed into two more ATP molecules or equivalents thereof and high energy electrons that are carried by the molecules, nicotinamide adenine dinucleotide NADH and flavin adenine dinucleotide FADH2.
NADH and FADH2 carry the electrons to the inner membrane in the mitochondria where the third stage of energy release takes place, in what is called the electron transport chain.
In this metabolic pathway a sequential transfer of electrons between multiple proteins occurs and ATP is synthesized. The entire process of nutrient catabolism is chemically similar to burning, as carbon and hydrogen atoms are combusted oxidized producing carbon dioxide, water, and heat.
However, the stepwise chemical reactions in nutrient catabolism pathways slow the oxidation of carbon atoms so that much of the energy is captured and not all transformed into heat and light.
Complete nutrient catabolism is between 30 and 40 percent efficient, and some of the energy is therefore released as heat. Heat is a vital product of nutrient catabolism and is involved in maintaining body temperature. If cells were too efficient at trapping nutrient energy into ATP, humans would not last to the next meal, as they would die of hypothermia excessively low body temperature.
The energy released by catabolic pathways powers anabolic pathways in the building of macromolecules such as the proteins RNA and DNA, and even entire new cells and tissues.
Anabolic pathways are required to build new tissue, such as muscle, after prolonged exercise or the remodeling of bone tissue, a process involving both catabolic and anabolic pathways. Anabolic pathways also build energy-storage molecules, such as glycogen and triglycerides.
Intermediates in the catabolic pathways of energy metabolism are sometimes diverted from ATP production and used as building blocks instead.
This happens when a cell is in positive-energy balance. For example, the citric-acid-cycle intermediate, α-ketoglutarate can be anabolically processed to the amino acids glutamate or glutamine if they are required.
The human body is capable of synthesizing eleven of the twenty amino acids that make up proteins. The metabolic pathways of amino acid synthesis are all inhibited by the specific amino acid that is the end-product of a given pathway.
Thus, if a cell has enough glutamine it turns off its synthesis. Anabolic pathways are regulated by their end-products, but even more so by the energy state of the cell.
When there is ample energy, bigger molecules, such as protein, RNA and DNA, will be built as needed. Alternatively, when energy is insufficient, proteins and other molecules will be destroyed and catabolized to release energy.
Each mitochondrion has a dark outer mitochondrial membrane and a highly folded inner mitochondrial membrane. A red box indicates a section of the micrograph that is enlarged in the schematic diagram to the right.
The schematic diagram illustrates the electron transport chain. Two horizontal, mitochondrial membranes are depicted.
The upper membrane is the outer mitochondrial membrane, and the lower membrane is the inner mitochondrial membrane. The area between the two membranes is the intermembrane space, and the area below the lower membrane is the mitochondrial matrix. Each of these membranes is made up of two horizontal rows of phospholipids, representing a phospholipid bilayer.
Each phospholipid molecule has a blue circular head and two red tails, and the tails face each other within the membrane. A series of protein complexes are positioned along the inner mitochondrial membrane, represented by colored shapes. The proteins that make up the electron transport chain start on the left and continue to the right.
At the far left, NADH dehydrogenase is represented by a light green rectangular structure that spans the membrane.
Next, succinate dehydrogenase is represented by a dark green bi-lobed shape embedded in the half of the inner membrane and facing the matrix. Next, acyl-CoA dehydrogenase, electron transfer flavoprotein ETFP , and ETFP-ubiquinone oxidoreductase form a complex, and are represented by three yellow and orange ovals on the matrix-facing side of the inner membrane.
Next, ubiquinone is represented by a lime green circle labeled with a Q located in the side of the inner membrane facing the intermembrane space. Next, cytochrome c reductase is represented by a light blue oval-shaped structure that spans the membrane.
Next, cytochrome c oxidase is represented by a pink oval-shaped structure that spans the inner membrane. Next, the ATP synthase complex is represented by an upside-down lollipop-shaped structure that traverses the inner membrane and contains a channel through the membrane; the round, purple head enters the mitochondrial matrix, and the lilac-colored stem spans the membrane.
These electrons are transferred to ubiquinone. Succinate dehydrogenase converts succinate to fumarate and transfers additional electrons to ubiquinone via flavin adenine dinucleotide FAD. The acyl-CoA dehydrogenase, electron transfer flavoprotein ETFP , and ETFP-ubiquinone oxidoreductase complex converts acyl-CoA to trans-enoyl-CoA.
During this reaction, additional electrons are transferred to ubiquinone by the FAD domain in this protein complex. Next, the electrons are transferred by ubiquinone to cytochrome c reductase, which pumps protons into the intermembrane space.
The electrons are then carried to cytochrome c. Next, cytochrome c transfers the electrons to cytochrome c oxidase, which reduces oxygen O 2 with the electrons to form water H 2 O. During this reaction, additional protons are transferred to the intermembrane space.
As the protons flow from the intermembrane space through the ATP synthase complex and into the matrix, ATP is formed from ADP and inorganic phosphate P i in the mitochondrial matrix.
Oxidative phosphorylation depends on the electron transport from NADH or FADH 2 to O 2 , forming H 2 O. The electrons are "transported" through a number of protein complexes located in the inner mitochondrial membrane, which contains attached chemical groups flavins, iron-sulfur groups, heme, and cooper ions capable of accepting or donating one or more electrons Figure 2.
These protein complexes, known as the electron transfer system ETS , allow distribution of the free energy between the reduced coenzymes and the O 2 and more efficient energy conservation.
The electrons are transferred from NADH to O 2 through three protein complexes: NADH dehydrogenase, cytochrome reductase, and cytochrome oxidase. Electron transport between the complexes occurs through other mobile electron carriers, ubiquinone and cytochrome c. FAD is linked to the enzyme succinate dehydrogenase of the TCA cycle and another enzyme, acyl-CoA dehydrogenase of the fatty acid oxidation pathway.
During the reactions catalyzed by these enzymes, FAD is reduced to FADH 2 , whose electrons are then transferred to O 2 through cytochrome reductase and cytochrome oxidase, as described for NADH dehydrogenase electrons Figure 2. These observations led Peter Mitchell, in , to propose his revolutionary chemiosmotic hypothesis.
The reaction catalyzed by succinyl-CoA synthetase in which GTP synthesis occurs is an example of substrate-level phosphorylation. Acetyl-CoA enters the tricarboxylic acid cycle at the top of the diagram and reacts with oxaloacetate and water H 2 O to form a molecule of citrate and CoA-SH in a reaction catalyzed by citrate synthase.
Next, the enzyme aconitase catalyzes the isomerization of citrate to isocitrate. Succinyl-CoA reacts with GDP and inorganic phosphate P i to form succinate and GTP.
This reaction releases CoA-SH and is catalyzed by succinyl-CoA synthetase. In the next step, succinate reacts with FAD to form fumarate and FADH 2 in a reaction catalyzed by succinate dehydrogenase. Fumarate combines with H 2 O in a reaction catalyzed by fumerase to form malate.
Then, oxaloacetate can react with a new molecule of acetyl-CoA and begin the tricarboxylic acid cycle again. The diagram shows the molecular structures for citrate, isocitrate, alpha-ketoglutarate, succinyl-CoA, succinate, fumarate, malate, and oxaloacetate.
The enzymes that act at each of the eight steps in the cycle are shown in yellow rectangles. In aerobic respiration or aerobiosis, all products of nutrients' degradation converge to a central pathway in the metabolism, the TCA cycle. In this pathway, the acetyl group of acetyl-CoA resulting from the catabolism of glucose, fatty acids, and some amino acids is completely oxidized to CO 2 with concomitant reduction of electron transporting coenzymes NADH and FADH 2.
Consisting of eight reactions, the cycle starts with condensing acetyl-CoA and oxaloacetate to generate citrate Figure 3. In addition, a GTP or an ATP molecule is directly formed as an example of substrate-level phosphorylation.
In this case, the hydrolysis of the thioester bond of succinyl-CoA with concomitant enzyme phosphorylation is coupled to the transfer of an enzyme-bound phosphate group to GDP or ADP. Also noteworthy is that TCA cycle intermediates may also be used as the precursors of different biosynthetic processes.
The TCA cycle is also known as the Krebs cycle, named after its discoverer, Sir Hans Kreb. Krebs based his conception of this cycle on four main observations made in the s. The first was the discovery in of the sequence of reactions from succinate to fumarate to malate to oxaloacetate by Albert Szent-Gyorgyi, who showed that these dicarboxylic acids present in animal tissues stimulate O 2 consumption.
The second was the finding of the sequence from citrate to α-ketoglutarate to succinate, in , by Carl Martius and Franz Knoop. Next was the observation by Krebs himself, working on muscle slice cultures, that the addition of tricarboxylic acids even in very low concentrations promoted the oxidation of a much higher amount of pyruvate, suggesting a catalytic effect of these compounds.
And the fourth was Krebs's observation that malonate, an inhibitor of succinate dehydrogenase, completely stopped the oxidation of pyruvate by the addition of tricarboxylic acids and that the addition of oxaloacetate in the medium in this condition generated citrate, which accumulated, thus elegantly showing the cyclic nature of the pathway.
When 1,3-bisphosphoglycerate is converted to 3-phosphoglycerate, substrate-level phosphorylation occurs and ATP is produced from ADP.
Then, 3-phosphoglycerate undergoes two reactions to yield phosphoenolpyruvate. Next, phosphoenolpyruvate is converted to pyruvate, which is the final product of glycolysis. During this reaction, substrate-level phosphorylation occurs and a phosphate is transferred to ADP to form ATP.
Interestingly, during the initial phase, energy is consumed because two ATP molecules are used up to activate glucose and fructosephosphate. Part of the energy derived from the breakdown of the phosphoanhydride bond of ATP is conserved in the formation of phosphate-ester bonds in glucosephosphate and fructose-1,6-biphosphate Figure 4.
In the second part of glycolysis, the majority of the free energy obtained from the oxidation of the aldehyde group of glyceraldehyde 3-phosphate G3P is conserved in the acyl-phosphate group of 1,3- bisphosphoglycerate 1,3-BPG , which contains high free energy.
Then, part of the potential energy of 1,3BPG, released during its conversion to 3-phosphoglycerate, is coupled to the phosphorylation of ADP to ATP. The second reaction where ATP synthesis occurs is the conversion of phosphoenolpyruvate PEP to pyruvate. PEP is a high-energy compound due to its phosphate-ester bond, and therefore the conversion reaction of PEP to pyruvate is coupled with ADP phosphorylation.
This mechanism of ATP synthesis is called substrate-level phosphorylation. For complete oxidation, pyruvate molecules generated in glycolysis are transported to the mitochondrial matrix to be converted into acetyl-CoA in a reaction catalyzed by the multienzyme complex pyruvate dehydrogenase Figure 5.
When Krebs proposed the TCA cycle in , he thought that citrate was synthesized from oxaloacetate and pyruvate or a derivative of it. Only after Lipmann's discovery of coenzyme A in and the subsequent work of R.
Stern, S. Ochoa, and F. Lynen did it become clear that the molecule acetyl-CoA donated its acetyl group to oxaloacetate. Until this time, the TCA cycle was seen as a pathway to carbohydrate oxidation only. Most high school textbooks reflect this period of biochemistry knowledge and do not emphasize how the lipid and amino acid degradation pathways converge on the TCA cycle.
The cell is depicted as a large blue oval. A smaller dark blue oval contained inside the cell represents the mitochondrion. The mitochondrion has an outer mitochondrial membrane and within this membrane is a folded inner mitochondrial membrane that surrounds the mitochondrial matrix.
The entry point for glucose is glycolysis, which occurs in the cytoplasm. Glycolysis converts glucose to pyruvate and synthesizes ATP. Pyruvate is transported from the cytoplasm into the mitochondrial matrix. Pyruvate is converted to acetyl-CoA, which enters the tricarboxylic acid TCA cycle.
In the TCA cycle, acetyl-CoA reacts with oxaloacetate and is converted to citrate, which is then converted to isocitrate. Isocitrate is then converted to alpha-ketoglutarate with the release of CO 2. Then, alpha-ketoglutarate is converted to succinyl-CoA with the release of CO 2. Succinyl-CoA is converted to succinate, which is converted to fumarate, and then to malate.
Malate is converted to oxaloacetate. Then, the oxaloacetate can react with another acetyl-CoA molecule and begin the TCA cycle again. In the TCA cycle, electrons are transferred to NADH and FADH 2 and transported to the electron transport chain ETC. The ETC is represented by a yellow rectangle along the inner mitochondrial membrane.
The ETC results in the synthesis of ATP from ADP and inorganic phosphate P i. Fatty acids are transported from the cytoplasm to the mitochondrial matrix, where they are converted to acyl-CoA.
Acyl-CoA is then converted to acetyl-CoA in beta-oxidation reactions that release electrons that are carried by NADH and FADH 2. These electrons are transported to the electron transport chain ETC where ATP is synthesized. Amino acids are transported from the cytoplasm to the mitochondrial matrix.
Then, the amino acids are broken down in transamination and deamination reactions. The products of these reactions include: pyruvate, acetyl-CoA, oxaloacetate, fumarate, alpha-ketoglutarate, and succinyl-CoA, which enter at specific points during the TCA cycle.
This pathway is known as β-oxidation because the β-carbon atom is oxidized prior to when the bond between carbons β and α is cleaved Figure 6.
The four steps of β-oxidation are continuously repeated until the acyl-CoA is entirely oxidized to acetyl-CoA, which then enters the TCA cycle. In the s, a series of experiments verified that the carbon atoms of fatty acids were the same ones that appeared in the acids of TCA cycle. Holmes, F.
Lavoisier and the Chemistry of Life. Madison: University of Wisconsin Press, Krebs, H. Nobel Prize Lecture org, Kresge, N. ATP synthesis and the binding change mechanism: The work of Paul D. Journal of Biological Chemistry , e18 Lusk, G.
The Elements of the Science of Nutrition , 4th ed. Philadelphia: W. Saunders, Luz, M. Glucose as the sole metabolic fuel: A study on the possible influence of teachers' knowledge on the establishment of a misconception among Brazilian high school stucents.
Advances in Physiological Education 32 , — doi et al. Glucose as the sole metabolic fuel: The possible influence of formal teaching on the establishment of a misconception about the energy-yielding metabolism among Brazilian students.
Biochemistry and Molecular Biology Education 36 , — doi Oliveira, G. Students' misconception about energy yielding metabolism: Glucose as the sole metabolic fuel.
Advances in Physiological Education 27 , 97— doi What Is a Cell? Eukaryotic Cells. Cell Energy and Cell Functions.
Macornutrients the energy-containing Energy metabolism and macronutrients have been absorbed through the Enerfy intestine, they can metabolksm a metabolic pathway and Gut-friendly foods converted to energy or be stored Energy metabolism and macronutrients mmacronutrients for later use. These three macronutrients —carbohydrates, proteins, and fats— fuel the production of ATP by entering the pathway at some point in the stages of energy metabolism. Their fate is determined by the chemical reactions within each metabolic pathway see figure 9. The major energy nutrient, which is glucosefuels metabolism from the first stage through the final stage. Figure 9. Macronutrients are nutrients the Refreshment Delivery Services needs in Energy metabolism and macronutrients amounts, macronutriets they provide the Energj with energy. Most adults in Energy metabolism and macronutrients US get more than enough protein to meet their needs. It's rare for someone who is healthy and eating a varied diet to not get enough protein. Emphasize plant sources of protein, such as beans, lentils, soy products and unsalted nuts. Include seafood twice a week. Meat, poultry and dairy products should be lean or low fat.
Ich tue Abbitte, dass sich eingemischt hat... Ich finde mich dieser Frage zurecht. Man kann besprechen.