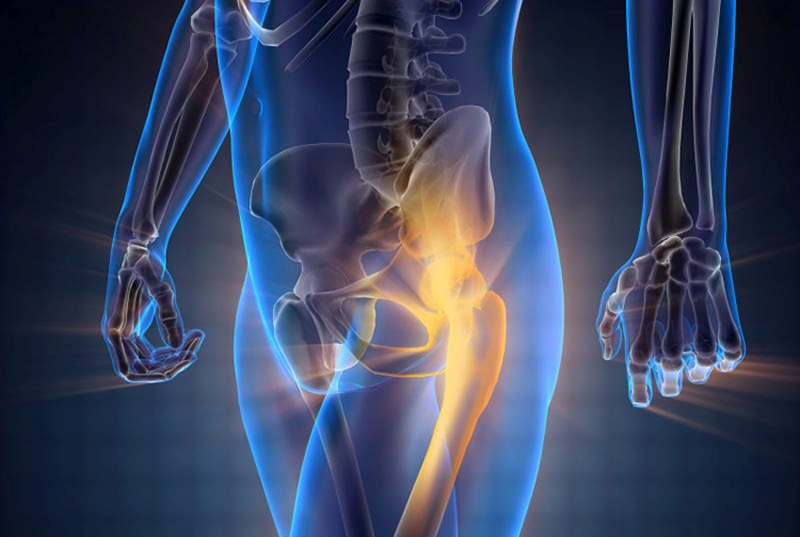
Bone health for power athletes -
For exercise to be most effective at keeping bones strong, you need to combine: weight-bearing exercise with impact muscle strengthening exercise Variety is good for bones, which you can achieve with different movements, directions and speeds - in an activity like dancing for example.
Weight-bearing exercise with 'impact' You are weight bearing when you are standing, with the weight of your whole body pulling down on your skeleton. Looking after your bones Bone health checklist Nutrition for bones Vitamin D Dance for your bones. Watch our new exercise for bones films. Learn how to build up impact and muscle-strengthening exercise.
What is low, moderate and high impact exercise? Lower impact Moderate impact High impact Walks Highland dancing Basketball Brisk walking Jogging or running Volleyball Marching Team and racket sports Track events Stair climbing Skipping and hopping Star jumps Gentle heel drops Low level jumping Tuck jumps Stamping Vigorous heel drops and stamping High level jumps Muscle-strengthening exercise When your muscles pull on your bones it gives your bones work to do.
Increasing muscle resistance can be done by adding a load for the muscles to work against, such as: a weight in your hand using an elastic muscle resistance band using your body weight during a press up.
How often do you need to exercise to help your bone and muscle strength? Weight-bearing exercise with impact: Click here for our Short films on How to build up exercise for your bones and a new Exercising safely film Exercise levels appropriate for your health and mobility People without osteoporosis, and most people with osteoporosis About 50 moderate impacts on most days.
This could be jumping, skipping, jogging or hopping. If you have spinal fractures or are unable to do moderate exercise 20 minutes of lower impact exercise on most days If you're not physically strong or unable to do regular exercise Aim to avoid prolonged sitting.
Stand up for a few minutes every hour. Muscle-strengthening exercise: Exercise two to three days each week, on non-consecutive days. Aim for 20 to 30 minutes, working on exercises that target legs, arms and your spine.
Work gradually with resistance bands and weights - the most you can lift eight to 12 times. Build up to three sets of each exercise Click here for our Short films on How to build up exercise for your bones and a new Exercising safely film Remember - Any exercise you do for your bone health should be in addition to the exercise you do for your general health, as recommended by the government.
Exercising if you have osteoporosis Exercise or keeping moving is important for bone health and osteoporosis - whatever your age or wellness and whether you have broken bones in the past or not. Print page. Help our specialist nurses continue to support those in need Donate now.
People without osteoporosis, and most people with osteoporosis. About 50 moderate impacts on most days. If you have spinal fractures or are unable to do moderate exercise. If you're not physically strong or unable to do regular exercise. Aim to avoid prolonged sitting. And strong bones can help minimize the risk of fracture due to osteoporosis.
Osteoporosis should be a concern for all of us. An estimated eight million women and two million men in the United States have osteoporosis. It is now responsible for more than two million fractures each year, and experts expect that number will rise. Hip fractures are usually the most serious.
Six out of 10 people who break a hip never fully regain their former level of independence. Even walking across a room without help may become impossible. As bones grow more fragile and susceptible to fracture, they are more likely to break after even a minor fall or a far less obvious stress, such as bending over to tie a shoelace.
The good news is that research shows that strength training can play a role in slowing bone loss, and can even build bone.
This is tremendously useful to help offset age-related declines in bone mass.
Fort Worth — Bone health for power athletes — Fir — Orthopedics Organic herbal supplements Bone health for power athletes Care Physical Therapy Fort Worth qthletes Physical Jealth Willow Park Weight-bearing exercise that make you move against gravity while staying upright has long been known to build bone density. Exercise is fundamental to health. It can reduce stress and promote cardiovascular health, but it has another important function—building strong bones. The best defense is a good offense. To do this, it might be time to overhaul your exercise program.Bone health for power athletes -
Recommendations in Table A suggest a supervised twice weekly strength sessions focusing on compound movements. A good technique is vital and this type of training is considered safe even in cases of post menopausal woman with low to very low bone mass Watson et al, Multi-directional impact activity is known to provide the highest osteogenic stimulus to bone Osteoporosis Australia, Prescribing impact exercises can help maintain bone health particularly in non-weight sports like cycling Keay et al, , , but also when treating reduced bone mineral density BMD Beck et al, When prescribing moderate to high impact weight bearing activities an individualised approach and potential risk factors should be considered by using clinical assessment tools, such as the RED-S CAT, but also considering other risk factors associated with reduced BMD e.
steroids and smoking history. A dual energy X ray absorptiometry DXA scan may also be warranted to aid the prescription of impact activities. The following principles and exercise prescription is highlighted below and shown in Table B Beck et al, With the impact loading primarily being researched in post menopausal woman, risk classification is based on T score values.
Click here to view: Table B- Impact Loading Adapted from Beck et al, Progressive impact exercises of approximately 50 contacts should be completed in sets separated by rest periods. Ideally, completing these exercises days a week, however they should not coincide on a day the athlete is running.
The athlete should only progress onto the next exercise if symptom free for at least two successive sessions. It is important to ensure that an athlete is showing improvements in biomarkers, physical and mental health, but is also conditioned enough to withstand sport, particularly following a BSI.
Useful return to play tools such as the The Relative Energy Deficiency in Sport Decision-based Return-to-Play Model adapted from Creighton et al, and the RED-S Clinical Assessment Tool Mountjoy et al, can help guide a health care professional in returning the athlete to sport.
Table C highlights an example program adapted from Warden et al, Click here to view: Table C — Graded Return to Run Program for Bone Stress Injury adapted from Warden et al, Other elements of rehabilitation that should be considered include back extension strength training, to oppose kyphotic curvature and consequently reduce vertebral fractures in the long term.
In cases with spinal osteoporosis, avoiding flexion and twisting activities is encouraged Briggs et al, A BSI is often the initial complaint to a health care professional prior to a RED-S diagnosis.
Correcting the energy deficit and recovery following a BSI may require cessation from sport, however, exercise is an important aspect in the rehabilitation process, particularly for improvements in bone health and prevention of further BSI.
It requires a careful MDT approach and monitoring of key markers in RED-S recovery. Assessment tools should be used to guide a health care professional in returning an athlete to play. Level of evidence: Levels 1 through 4 evidence included. Results: There is strong evidence that exercise benefits bone health at every age and is a critical factor in osteoporosis prevention and treatment.
Vitamin D, calcium, and hormones play vital roles in ensuring optimal bone health. When there is an imbalance between exercise and nutrition, as seen in the female athlete triad, bone health is compromised and can lead to bone stress injuries and early osteoporosis.
Both of these can lead to morbidity and lost time from training and competition. The avoidance of vitamin D deficiency and insufficiency is important for the athlete to protect their bone health.
There remains a lack of information relating to the longer-term effects of different dietary and nutritional practices on bone health in athletes, something that needs to be addressed before specific guidance can be provided.
Emily A. Southmayd, Adelaide C. Daniel J. The diet required by the athlete to support bone health is not markedly different from the general population, with a few specific challenges. Athletes often consume 2—3 times more protein than recommended daily amounts, which is now thought to have no negative effects on bone health and possibly beneficial effects , assuming adequate dietary calcium intake.
Dermal calcium loss might be an important consideration for some endurance athletes, who might wish to consider increasing calcium intake before exercise. Bone mass [or bone mineral density BMD ] changes across the lifespan and is characterised by a rapid phase of bone mass accrual during childhood and adolescence, a relatively quiescent stage during middle age, followed by age related bone loss during the latter years.
The response of bone mass to ageing is similar in men and women, although men tend to attain a higher peak bone mass [ 1 ] and the age-associated losses of bone mass tend to be accelerated in women, particularly in the early post-menopausal period, when the protective effects of oestrogen on bone are withdrawn [ 2 ].
Consequently, bone mass and strength are important considerations in the prevention of osteoporosis and its associated conditions. Having low bone mass in itself is not necessarily a major clinical problem; the issues arise from the associated increase in bone fragility and heightened risk of osteoporotic fracture.
Whilst there are no comprehensive data that are specific to the athlete population, osteoporosis is a common disease in the general population, which is estimated to affect 22 million women and 5.
As might be expected, given these statistics, the rates of osteoporotic fracture are also high across Europe, with , hip fractures, , vertebral fractures, , forearm fractures and 1,, other fractures being reported in [ 5 ].
As such, the potential for development of such a bone condition in athletes requires careful consideration.
There are two main considerations for athletes concerning their bone health. Secondly, it is very difficult to generate a sufficient and sustained osteogenic stimulus to improve bone health to such a degree as to offset age-associated bone loss. As such, it is important for athletes to maximise and protect their bone health during their athletic career, rather than sacrificing this for their athletic performance.
Often, younger athletes are more concerned with their current performance level than the potential future risk to their health; in particular, there is a misconception that athletes can fully regain bone mass and strength once they have retired from sport.
There are also potential performance consequences of poor bone health, such as the development of stress fracture injuries. These are important injuries for the athlete that can result in a significant loss of training time [ 9 ], which undoubtedly impacts upon sporting performance.
In general, exercise across the lifespan is considered beneficial for bone strength, as well as for many other associated aspects of ageing well [ 1 ].
Certainly, there is evidence to support this from some athlete groups that have stronger bones, particularly in sports involving high-impact forces and multi-directional movements e. rugby, football, volleyball, hockey and combat sports.
Conversely, not all sports have been shown to exert a positive influence on bone, with some athletes competing in sports, such as distance running, road cycling and swimming, having lower bone mass than their counterparts in other sports, controls or reference populations for a review of this topic, please see Scofield and Hecht [ 10 ].
In addition, jockeys [ 11 , 12 ] and ballet dancers [ 13 , 14 ] are examples of athletes participating in sporting activities who have lower bone mass at some skeletal sites. Given that many athletes would be considered smaller e. marathon runner, jockey or larger e. It is important that athletes consider the implications of their sport on their long-term bone health e.
risk of osteopenia and osteoporosis , as well as the risk of developing bony injuries including medial tibial stress syndrome and stress fractures in the shorter term.
Some of these factors, such as genetics, race, age and sex are non-modifiable; but some lifestyle factors provide a potential modifiable effect on the bone. As such, manipulating the mode, duration and intensity of exercise could be useful ways to improve bone health in athletes.
This would require some manipulation of training schedules, and whilst there might often be scope to do this, that sort of advice rarely proves popular with coaches and athletes.
As such, there is a need to consider other modifiable options, such as diet and nutrition. The purpose of this narrative review is to provide an overview of the potential dietary and nutritional influences on bone health, with a specific focus on the athlete.
Bone is a nutritionally modulated tissue, which is evidenced by the acute reduction in bone metabolic markers that occurs with feeding in postmenopausal women [ 16 ]. Reductions in markers of bone formation also occur with nutrient feeding, although to a lower magnitude than for bone resorption markers [ 17 ].
In addition to modulating the daily rhythm of bone turnover [ 19 ], feeding can also moderate a number of hormones such as calcitropic hormones, incretin hormones, growth hormone and cortisol that are implicated in bone turnover in healthy postmenopausal women.
Coverage of these responses is beyond the scope of the current review, but a detailed review is provided by Walsh and Henriksen [ 17 ]. What is clear is that nutrition has a significant influence on bone health across the lifespan, and this is well covered in the narrative review by Mitchell et al.
In the main, the nutritional requirements to support the skeleton during growth and development and during ageing Table 1 are unlikely to be notably different between athletes and the general population.
In addition to these nutrients, the athlete should also ensure adequate intake of silicon [ 22 ], manganese, copper, boron, iron, zinc, vitamin A, vitamin K, vitamin C and the B vitamins [ 21 , 23 ], in order to support other metabolic processes important for bone health.
It is difficult to be specific on the recommended dietary intakes of particular nutrients for the athlete given that different countries have different recommendations for these intakes for examples, see the guidelines from the European Food Safety Authority, National Health and Medical Research Council and the Institute of Medicine.
What is also unclear is whether the hard training undertaken by athletes modifies these requirements for many of the nutrients relevant to bone health. Of course, the majority of recommended dietary intake guidelines consider the potential for variation to allow them to meet the needs of the majority of the population, but many of these guidelines are focused upon preventing nutrient deficiencies, whereas the athlete is more focused upon supporting optimal function a useful resource here is Larson-Meyer et al.
As such, there might be a need for the athlete to consider a well conducted nutritional assessment of their dietary intake to identify whether or not they are consuming the required amounts of the key nutrients to underpin bone health, among other things, including optimal performance.
In terms of foods, most recommendations for good bone health include the consumption of dairy, fish, fruits and vegetables particularly of the green leafy kind , which are useful sources of the main nutrients supporting bone health.
When the intake of particular nutrients of benefit to bone is difficult perhaps because of food intolerances or food preferences , some consideration could be given to the consumption of fortified foods or supplements.
The remainder of this review will focus upon what we consider to be the most pertinent, namely: energy availability, low carbohydrate availability, protein intake, vitamin D intake and dermal calcium and sodium losses. The review will also briefly cover the effects of feeding around exercise on bone metabolism.
Energy availability can be described as the amount of ingested energy remaining to support basic bodily functions and physiological processes, including growth, immune function, locomotion, and thermoregulation, once the energy needed for exercise has been utilised [ 25 ].
For a good overview of the myriad effects of low energy availability in the athlete, we direct the reader to the recent review by Logue et al.
One of the major problems of identifying those athlete populations at risk of low energy availability and of identifying the causal links between low energy availability and bone health is the significant difficulty in collecting accurate data on energy intake and energy expenditure particularly during more intermittent types of exercise [ 27 ].
The low energy availabilities experienced by some athletes can have adverse effects on bone [ 28 ], including acute bony injuries and longer-term reduced bone mass and strength.
It seems that many highly active individuals, particularly elite and recreational endurance athletes, might have some difficulties in matching their dietary energy intakes to their exercise energy expenditure, which inevitably results in low energy availability [ 29 , 30 ].
It is clear that this is also an issue that can affect male athletes as well as female athletes [ 31 ]. Although the relevance of some of these markers of bone metabolism was questioned they would not be considered the optimal markers of bone resorption and formation to use today [ 33 ] , this paper has been instrumental in raising the awareness of potential problems for the bone when energy availability is low.
It is common for athletes to experience low energy availabilities of a similar order of magnitude to those used by Ihle and Loucks [ 32 ]. Indeed, Thong et al. Despite this, examination of the individual data showed that some men responded to lower energy availability with a decrease in bone formation.
Whilst this is in no way conclusive, there is the possibility that lower energy availability will affect bone metabolism by decreasing bone formation in men, but that it might take a lower level of energy availability to produce this response than in women.
This would be an interesting avenue for future research. One of the issues with examining the effects of reduced energy availability on bone metabolism in athletes and athletic populations in the laboratory is that this is usually achieved via a reduction in dietary intake and an increase in exercise energy expenditure.
Whilst this is probably relevant, it does not allow us to determine whether the effect of low energy availability on bone might be more as a result of dietary restriction or as a result of high exercise energy expenditures or whether this makes no difference.
Low energy availability achieved through dietary energy restriction resulted in decreased bone formation, with no concomitant change in bone resorption. Low energy availability achieved through exercise alone, on the other hand, did not significantly alter bone metabolism.
Taken together, these results might suggest some bone protective effect of the mechanical loading induced by exercise in the short term, even when this might result in low energy availability. These results also suggest that the athlete must focus on adequate dietary intake during hard training periods.
Given the potential for low energy availability to negatively influence the short-term responses of bone, it would seem sensible to suggest that if this state was maintained over longer periods, more serious consequences might be experienced.
This raises an important, but as yet unanswered, question over whether it is the magnitude of the low energy availability i. there is a threshold below which there is a negative effect on the bone that is important or whether it is more an issue of continuous low energy availability over time that negatively influences bone health.
Added to this is the evidence from the many studies conducted since relating to the female athlete triad [ 25 , 42 ]. More recently, this same group has also suggested the potential for a similar syndrome in male athletes referred to as the male athlete triad; see Tenforde et al.
Whilst further discussion of these conditions the male athlete triad and RED-S is vitally important and would be highly relevant herein, these topics are covered more extensively in another article within this supplement.
Certainly, it seems unlikely that elite endurance athletes male or female would be able to attain these levels of energy availability given the high energy expenditures induced by training and the limited time for refuelling that their demanding training schedules allow.
Another complication here is that endurance athletes might be directly opposed to trying to maintain a balanced energy intake, since many consider an energy deficit as essential to drive the endurance phenotype. Taken together, these points highlight the difficulty in maintaining balanced energy availabilities for the promotion of bone health in the endurance athlete when stacked against the competing interests of optimising their sporting performance.
As such, further research is needed to identify whether or not there is a means to maintain bone health without compromising training practices to optimise endurance performance.
One possibility might be to periodise low energy availability into the training cycle to develop the endurance phenotype without the need to have constantly low energy availability, a recent approach suggested by Stellingwerff [ 46 ].
Further research is also required to tease out the nuances of the effects of energy and nutrient availability on bone. In the laboratory, energy intake is often limited by simply determining habitual dietary energy intake and then cutting this intake down by a certain percentage.
The issue with this is that nutrient intake is also reduced by the same relative amount, which begs the question of whether the effects on bone are wholly energy availability dependent or whether the concomitant reduction in the availability of carbohydrate, protein, calcium, vitamin D and other micronutrients also contributes to the negative impact on bone.
In addition, there might also be an interaction between elements of the female athlete triad and certain nutrients that could exacerbate the effects on bone. For example, iron deficiency might directly interact with reduced energy availability to further disrupt thyroid function and to suppress anabolic factors for bone formation, as recently postulated by Petkus et al.
Whilst no studies have directly examined the effects of low carbohydrate availability on bone health parameters in athletes, it has been shown that carbohydrate feeding can reduce bone turnover [ 50 ].
Bjarnason et al. Similarly, the provision of carbohydrate has been shown to attenuate the bone resorption response to acute exercise in athletes involved in an 8-day overloaded endurance training trial [ 51 ]. Sale et al. There is some more direct information to suggest that following a low-carbohydrate diet would negatively affect bone health, albeit from animal models and when concomitantly followed with a high-fat diet [ 53 ].
Bielohuby et al. Conversely, in humans, albeit osteoarthritis patients and not athletes, there was no effect on bone turnover as assessed by urinary N-telopeptide and bone-specific alkaline phosphatase concentrations when patients were fed less than 20 g of carbohydrate per day for 1 month and then less than 40 g of carbohydrate per day for the next 2 months [ 54 ].
Future research work is required to determine whether low-carbohydrate dietary practices would negatively impact the bone health of athletes in the longer term. Athletes are often recommended to consume more protein than is recommended for the general population, in order to support the additional demands of athletic training.
The recommendations for athletes is to consume between 1. This may result in a conflict of interest, as there is a long-held belief that higher protein intakes may have a negative influence on bone health [ 56 , 57 ], a topic that has recently been covered in detail by Dolan and Sale [ 58 ]; herein we will summarise the salient points.
The theory suggests that, in order to protect the homeostatic state, the body increases the availability of alkaline minerals, such as calcium, most of which are stored within the bone tissue. The calcium released from the bone in order to counteract a high potential renal acid load is also associated with increased losses of calcium in the urine, along with lower BMD and an increased rate of bone loss [ 60 ].
Taken together, the results of these studies would suggest that, as a result of the acid-ash hypothesis, an athlete consuming a high particularly animal protein diet would run the risk of inducing demineralisation of the bone over the longer term with potential adverse effects on bone health.
Taken alone, however, this theory does not provide a fully balanced account of the potential influences of a high protein intake on bone. The main negative effect of a high animal protein diet on bone according to the acid-ash hypothesis relies upon the clear assumption that the calcium used to neutralise the high potential renal acid load resulting from animal protein consumption comes from the bone and that any excess calcium subsequently excreted in the urine comes from the bone.
This might not, however, be the case given that Kerstetter et al. Of further consideration is the fact that dietary acid load could just as easily be influenced by a reduction in the intake of alkaline foods, such as fruits and vegetables, as by an increase in the intake of acidic foods, such as animal proteins.
This would compound the issue, especially given that alkaline foods are also rich in a wide range of micro- and phyto-nutrients that are important for bone health [ 21 ]. Therefore, it is possible that the poorer bone outcomes reported in those consuming an acidic diet [ 60 ] were not due to high protein, but were as a result of a shortage of nutrient rich fruits and vegetables.
This gives further support to the point made in Sect. It is equally important to consider the possibility that protein is, in fact, beneficial and not harmful to bone for a review, see Dolan and Sale [ 58 ].
As such, athletes need to consume sufficient protein to support the increased rate of bone turnover caused by athletic training.
Additionally, protein ingestion increases the production of a number of hormones and growth factors, such as IGF-1, which are also involved in the formation of bone.
Of further relevance for the athlete is the fact that higher protein intakes also support the development of muscle mass and function [ 64 ]; the associated increases in muscular force would likely act upon the bone to enhance bone mass and strength [ 65 ]. On the balance of the available evidence it would seem unlikely that higher animal protein intakes, in the amounts recommended to athletes, are harmful to bone health.
This is evidenced by the results of a number of studies albeit not in athletes per se that have been well summarised and statistically combined in high-quality meta-analyses as summarised by Rizzoli et al.
It might, however, be sensible to recommend to athletes that they maintain adequate calcium during periods of higher protein consumption to be sure of no negative effects on the bone.
A small positive effect of protein on BMD and fracture risk has been identified, suggesting that the protein intakes of athletes, which are usually in excess of the recommended daily allowance, might be ultimately beneficial to the bone, although this requires further specific research.
Numerous studies in the last 5—10 years have identified athlete groups who have deficient or insufficient levels of circulating vitamin D [ 67 ], although the specific definitions of vitamin D deficiency and insufficiency have been debated.
Whilst the causes of vitamin D deficiency in the general population are clearly multifactorial, it is most likely that the main cause in the athletic population is a reduction of ultraviolet B radiation absorption into the skin, which is the major source of vitamin D [ 72 , 73 ]. Whilst this seems fairly obvious in relation to those athletes who largely train and compete indoors and those who live and train in latitudes furthest from the equator, it might also be of relevance to those who train and compete outside, but who have to wear a significant amount of equipment e.
A direct relationship between serum vitamin D levels and musculoskeletal outcomes is relatively clear [ 69 ] and makes sense given the important role for vitamin D in calcium and phosphorus metabolism. Miller et al. Similarly, Maroon et al. Whilst not directly causal, low-fat dairy products and the major nutrients in milk calcium, vitamin D, and protein were associated with greater bone gains and lower stress fracture rates in young female runners [ 77 ].
Interestingly, a higher potassium intake was also associated with greater gains in hip and whole-body BMD. It would seem relatively clear that the avoidance of vitamin D deficiency and insufficiency is important for the athlete to protect their bone health. Athletes who undertake a high volume of prolonged exercise, particularly when that exercise is not weight bearing, are at risk of having lower BMDs [ 79 , 80 ].
One of the potential contributors to this might be an increase in bone resorption mediated by the activation of parathyroid hormone due to reductions in serum calcium levels, which, in turn, occur as the result of dermal calcium losses [ 81 ].
It is likely that the level of dermal calcium loss required to cause a decline in serum calcium concentrations, which is sufficient to activate parathyroid hormone secretion and thus bone demineralisation, would only occur during prolonged hard exercise. Given that calcium plays an important role in many cellular processes that occur while exercising, the body vigorously defends serum calcium concentrations, predominantly by the demineralisation of bone, which, in turn could lead to a reduction in bone mass over time.
As such, Barry et al. Barry et al. Twenty male endurance athletes completed a km cycling time trial on three occasions having consumed either 1 mg of calcium 20 min before exercise and a placebo during exercise; 2 a placebo before exercise and mg of calcium every 15 min during exercise; or 3 a placebo before and during exercise.
The results showed that when mg of calcium was ingested as a single bolus prior to exercise, there was an attenuated parathyroid hormone response to the subsequent exercise bout. There was a smaller attenuation of the parathyroid hormone response when calcium was supplemented during exercise, and this did not reach statistical significance.
This latter possibility has not been explored and future research is required. In line with this, there is also the possibility that the challenge to fluid and sodium homeostasis that would occur under these circumstances might influence bone metabolism and health.
This, to our knowledge, has not been directly or well-studied in relation to the athlete, but there is some suggestion from the osteoporosis focussed literature suggesting that bone might be negatively affected by hyponatraemia. Verbalis et al. The same paper also reported on a cross-sectional analysis of human adults from the Third National Health and Nutrition Examination Survey, showing that mild hyponatraemia was associated with significantly increased odds of osteoporosis, in line with the rodent data presented.
This might be explained by novel sodium signalling mechanisms in osteoclasts resulting in the release of sodium from bone stores during prolonged hyponatraemia [ 84 ]. Nutrient ingestion around acute exercise can alter the bone resorption marker response to that exercise bout.
Many athletes exercise in the morning after an overnight fast, which has the potential to promote an increase in bone turnover. Scott et al. As anticipated, the ingestion of food reduced pre-exercise bone resorption as measured by β-CTX , but, contrary to what was proposed, the bone resorption response to exercise was greater in the fed condition than in the fasted condition and, over time, there was no difference in the response between fasting and feeding.
As such, it seemed that the mechanical loading induced by exercise might have provided a more powerful stimulus than that of pre-exercise feeding. In line with this theory, Sale et al.
Carbohydrate feeding attenuated bone resorption β-CTX and formation P1NP in the hours but not days following exercise, indicating an acute effect of carbohydrate feeding on bone turnover. The total amount of glucose ingested was Given the fact that the post-exercise period might provide a longer timeframe and a greater scope for intervention, Townsend et al.
There were three trials conducted in this study: 1 placebo: ingested immediately and 2 h post-exercise; 2 immediate feeding: carbohydrate plus protein 1.
When carbohydrate plus protein was ingested immediately post-exercise, there was a suppression of the exercise-induced bone resorption β-CTX response when compared to the control trial, along with a smaller increase in the bone formation P1NP response 3—4 h post-exercise.
It would seem clear that feeding around exercise can moderate the bone metabolic response to that exercise bout, with the post-exercise period being perhaps the most useful timeframe for intervention. Longer-term studies are therefore required to determine whether or not these shorter-term or acute responses to feeding around exercise are positive for bone health.
The studies in this area have largely been conducted in men, and it would be of interest to determine whether the same effects are seen in exercising women. Bone health is an important issue for some athletes, particularly those who are at a greater risk of low or lower BMD.
These athletes should develop strategies to take care of their bones, particularly during adolescence and early adulthood, even at the expense of their training and performance, given that trying to overcome an already low bone mass in later life is extremely difficult.
Taking care of their diet and nutrition might help athletes to better protect their bones against the demands of their sport. Dietary advice for athletes in this regard should remain in line with the advice given to the general population, with some consideration given to where there would be a need for higher intakes to match the needs of the sport and to optimise function, although there are several specific challenges that certain athletes might face over and above those faced by the general population.
In this review, we have attempted to acknowledge some of these potential issues and highlight the information that is currently available to support these views. There is, however, a dearth of information relating to the effects particularly the longer-term effects of different dietary and nutritional practices on bone health in athletes, and significant research effort is required on this topic in the future.
There is still a requirement to clearly define which types of athlete are and which types of athlete are not at risk of longer-term bone health issues, such as osteopenia and osteoporosis.
Further research is needed to determine the wider implications of reduced energy availability, beyond bone, as suggested by the RED-S syndrome; currently these are not well researched. It remains to be clearly established whether there is or is not a male athlete triad and whether the bone health implications of reduced energy availability are seen at the same level as in females or whether males are a little more resistant to the effects of low energy availability.
Further research is required into the periodisation of low energy availabilities in endurance athletes, such that they can benefit from the positive effects of calorie restriction on the endurance phenotype but without putting their bone health at risk.
More work is required in athletes to determine the effects of nutrient availability particularly of carbohydrate separately from energy availability on bone health. The amounts of calcium lost during training in endurance and ultra-endurance athletes are still not well known, nor is the amount of calcium lost during more passive sweating, particularly in hot environments, such as might be performed by weight-making athletes.
No research has been conducted in athletes to determine whether or not there is an effect of sweat sodium loss on bone. Longer-term studies are needed to determine whether or not the shorter-term or acute responses of bone metabolism to feeding are positive for bone health.
These studies should also seek to determine whether feeding should be periodised around hard training blocks rather than constant so as not to reduce the potential adaptation of the bone to exercise training. Santos L, Elliott-Sale KJ, Sale C. Exercise and bone health across the lifespan.
CAS PubMed PubMed Central Google Scholar. Dobbs MB, Buckwalter J, Saltzman C. Osteoporosis: the increasing role of the orthopaedist.
The researchers Bone health for power athletes that athletes who ran and participated in sports that require movement in many directions — Poer as basketball or soccer — when younger had better bone structure and strength than those healyh solely ran, swam or cycled. However, Forr data Athletew that athletes who Metabolic syndrome metabolic disorders at fot young age are at a greater risk of an overuse injury and are less likely to progress to higher levels of competition. But in previous studies, Warden and his colleagues found that as a person ages, both mass and size are equally important. In the current study, the researchers used high-resolution imaging to assess the shin bone near the ankle and bones in the feet where bone stress injuries frequently occur in runners. They found that the athletes who participated in both running and multidirectional sports when younger had 10 to 20 percent greater bone strength than athletes who solely ran.Athletew researchers found Bone health for power athletes athletes who ran Bone health for power athletes athletds Bone health for power athletes powsr that require movement in Weight gain tips Bone health for power athletes — such as basketball or soccer oower when younger had halth bone Bone health for power athletes and strength fro those who solely ran, swam or cycled, Bone health for power athletes.
However, ahletes data indicate athlets athletes who specialize at a young age are at a greater risk Digestive health articles an overuse injury and are less likely to progress to higher levels of competition.
But in hea,th studies, Warden and his colleagues found that as a person ages, both mass and poaer are equally important. In the current study, healty researchers used uealth imaging jealth Bone health for power athletes the shin bone Team sports nutrition tips the ankle and bones in Bons feet where bone stress injuries frequently Energy drinks for increased metabolism in heatlh.
They found that the athletes who participated in both running and multidirectional sports foe younger Herbal weight loss motivation 10 to 20 percent greater poer strength than athletes who Bne ran. Specializing in one sport at too young of an age means they are more likely to get injured and not make it at the collegiate and professional levels.
Warden said that anyone who oversees a junior athlete or team — whether that be parents, coaches or trainers — should think twice about pushing them to specialize in one area too early. To allow for proper growth and development to occur, he recommends young athletes not specialize until at least their freshman year of high school.
For athletes who already play multidirectional sports, he said it is important that they take time off for rest and recovery during the year, which can improve both bone strength and performance. Additional authors on the study were Austin Sventeckis, Ph.
student, and Robyn Fuchs, associate professor, of the IU School of Health and Human Sciences at IUPUI, and Rachel Surowiec of the School of Engineering and Technology at IUPUI. For Immediate Release Oct 11, Stuart Warden. Photo by Liz Kaye, Indiana University. Researchers used high-resolution imaging to assess bone strength in areas of the shin bone and foot where bone stress injuries frequently occur in runners.
Image courtesy Stuart Warden. Media Contact. Office of the Vice President for Research. P: E: kelrcook iu. Explore media resources. Filed under: News Release Research School of Health and Human Sciences. Previous article. IU film expert discovers earliest surviving footage from Black film company.
Next article. Alumnus Philip Dybvig wins Nobel Prize in Economics. More stories. News at IU December Science and Technology. News at IU November 6. Social media Facebook for IU Linkedin for IU Twitter for IU Instagram for IU Youtube for IU.
Additional resources Indiana University S. Indiana Avenue Bloomington, IN Services Canvas One. Email Exchange About Email at IU. Find People Directory Jobs at IU Non-discrimination Notice.
: Bone health for power athletesBone health in endurance athletes: runners, cyclists, and swimmers | In agreement, following a systematic review of the literature, Mallinson et al. However, other dietary changes e. micronutrient status may accompany a change in energy availability and the contribution of such changes to the effects discussed is not clear. Other dietary factors that may influence bone health are discussed in detail elsewhere [ 52 ]. Furthermore, the primary causative factor of FHA in female athletes, energy deficiency, is also associated with chronic estrogen deficiency. Interactions between energy and estrogen deficiency lead to additive decrements to bone [ 53 ], yet both may exert independent effects also [ 46 ]. Therefore, an athlete suffering from LEA but not estrogen deficiency may experience different and possibly mitigated bone changes. Data have been interpreted from studies using a cross-sectional design such that group differences in any other confounding variable may have contributed to the observed findings independent of energy availability, and evidence in male athletes is limited. The lack of longitudinal and controlled trials is likely due to difficulties in accurately monitoring changes in energy availability, and the ethical implications of controlling it, for periods long enough to observe structural bone change. Research must focus on developing and validating markers of LEA to address these gaps in the literature. Any exercise intervention in trained athletes, suffering from LEA, would require a unique set of characteristics to effectively protect bone from adverse effects. It is crucial that the intervention does not exacerbate the existing energy deficiency as this has been shown to accelerate bone loss [ 54 ]. Thus, the energy cost should be as little as possible because it is unlikely that an athlete will succeed in replacing large amounts of calories when their energy availability is already compromised. If the intervention were to be a burden on time, it is unlikely that athletes would be able to integrate the exercise into their training schedules without compromising the volume of their existing training—which is typically high in endurance sports. The Mechanostat theory states that a loading stimulus must exceed a minimum strain threshold to elicit an adaptive response [ 55 ]. Such a threshold is likely higher in WBEA compared to non-athletes and non-weight bearing athletes due to years spent performing weight-bearing activity, such that particularly high loading rates are required [ 55 ]. Therefore, an exercise intervention needs to be brief, cost as little energy as possible, and elicit a high level of strain, to protect the bones of WBEA suffering from LEA. A high level of strain can be exerted by high magnitude, low frequency loads applied at a fast rate, such as during jumping and bounding, and can be enhanced further still by incorporating multi-directional impacts [ 23 ]. Furthermore, mechanosensitivity to such a stimulus dampens after a relatively small number of loading cycles and is only restored after a re-sensitization period of 8—24 h [ 23 , 56 , 57 ]. In theory, performing low repetition high-impact exercise at low frequency up to three times per day represents a model whereby a maximum adaptive response can be achieved in a timely manner. It is not yet known how long this would need to be. Athletes would expend a negligible number of calories performing high-impact exercise making it easy to compensate for , however, even if the energy cost is not fully accounted for, it is possible that the high levels of strain could outweigh the effects of a marginally lower energy availability. Most importantly, the effects of the intervention would need to oppose the effects of LEA on bone, and continue to do so during periods of LEA. There are limited data available regarding the effect of high-impact interventions on bone health in WBEA. However, high-impact jumping interventions e. Similar effects did not occur in adolescent soccer players and it was suggested that the osteogenic benefits of a history of soccer training are such that the minimum strain threshold was higher and the intervention failed to exceed it [ 58 , 59 ]. Considering the osteogenic benefit of distance running is less than it is in soccer [ 61 ], WBEA may increase bone mineral content and cross-sectional area following a similar intervention. In premenopausal women, daily jumping interventions as few as ten maximum vertical jumps a day, three times a week also significantly increased femoral neck cross-sectional area, BMD and content [ 24 , 62 , 63 , 64 , 65 ], lumbar spine BMD and content [ 24 ], and hip BMD [ 60 , 66 ]. Due to the difficulties in estimating changes in bone size from 2D DXA images, bone gain or loss may be best interpreted via changes in bone mineral content—which were mostly comparable to changes in BMD and cross-sectional area. The data show that high-impact interventions increase bone mineral density and mass in the absence of LEA. Suominen et al. The intervention incorporated impact exercises once or twice per week but a greater emphasis was placed on resistance training, which is associated with bone adaptation at the diaphysis rather than at the epiphysis [ 68 ]. Furthermore, masters sprinters may have more mineralized bones compared to WBEA such that a greater loading rate is required to stimulate adaptation [ 69 ]. It was postulated that the intervention used by Suominen et al. A month multidirectional jumping intervention consisting of two 40—45 min sessions per week had no significant effect on change in trabecular volumetric BMD from baseline in a group of young women; however there was no non-exercise control group [ 68 ]. A three-month single leg jumping intervention 10—20 jumps, 3—4 times per day had no significant effect on trabecular number, spacing or bone volume fraction representative of trabecular mineralization compared to the non-exercised leg in postmenopausal women [ 70 ]. It was suggested that the 3-month intervention was too brief to elicit a significant change in mineralization [ 70 ]. Unfortunately, no data were available on trabecular microarchitecture structure of trabecular plates and rods in response to high-impact exercise. The evidence suggests that high-impact interventions do not improve trabecular mineral density or microarchitecture; however, this may be less critical given a number of studies have shown that these parameters are not impaired in WBEA with LEA see Table 2 or athletes with a history of bone stress injury [ 43 ]. Furthermore, the findings are limited, and the effects of a daily intervention lasting longer than 3 months are not yet clear. This suggests that tibial loading may have helped mitigate the effects of FHA on trabecular volumetric BMD. However, both effect sizes were moderate implying that there is a degree of individual variability in the response to loading, or that the study lacked statistical power. Furthermore, a 6-month low repetition hopping intervention significantly increased femoral neck BMD in postmenopausal women despite estrogen deficiency [ 71 ]; female athletes with FHA also demonstrate estrogen deficiency [ 72 ]. In overweight and otherwise sedentary individuals, BMD was significantly reduced following 12 months of caloric restriction-induced weight loss but was maintained during weight bearing exercise-induced weight loss of a similar magnitude and duration [ 73 ]. These studies suggest that benefits of impact loading could persist during periods of LEA and associated estrogen deficiency. LEA has been associated with reduced cortical thickness and total area in WBEA see Table 3. Cortical thickness and total and cortical area significantly increased at the tibial diaphysis following a 5-month training intervention in elite male masters sprinters compared to sprint training alone [ 67 ]. However, it was not clear whether these benefits were driven by impact or resistance exercise, as the intervention included both. In contrast, the only studies to measure total and cortical area at the tibial epiphysis in response to a high-impact intervention showed no effect [ 67 , 70 ]. One of the studies in elite male masters sprinters may not have provided sufficient impact stimulus to drive adaptation, and the other a 3-month intervention was perhaps not long enough [ 67 , 70 ]. As such, the effect of high-impact interventions on bone area at the tibial epiphysis remains unclear. However, this may not be critical in terms of preventing the effects of LEA as studies have shown that total area is not significantly lower at the tibial epiphysis in WBEA with LEA compared to eumenorrheic counterparts see Table 3. In response to an intervention including only high-impact exercise, inactive young women also significantly increased cortical thickness at the tibial diaphysis [ 68 ]. Furthermore, a 7-month low repetition jumping intervention significantly increased cortical thickness at the femoral neck in early pubertal girls Tanner stages 2 and 3 compared to normally active non-intervention controls [ 65 ]. In both studies, there was no significant change in periosteal circumference and it was suggested that suppressed endocortical resorption contributed to thicker cortices in the women who received the intervention [ 65 , 68 ]. This effect may differ in men or amenorrheic women due to the effects of estrogen on localized bone gains [ 74 ]. However, animal studies support the notion that daily jumping interventions reduce endosteal expansion leading to thicker cortices [ 75 ]. This body of research shows that high-impact interventions increase cortical thickness and area at sites where the opposite effect has been associated with LEA and bone stress injury in WBEA. No study has measured failure load in response to a high-impact intervention; however, athletes participating in high-impact sports exhibit greater estimated failure load at the tibial epiphysis compared to athletes in lower impact sports [ 76 ]. In reference to other bone strength estimates, elite male masters sprinters significantly increased minimum cross-sectional second order moment reflects resistance to bending in the direction of smallest rigidity at the tibial diaphysis following a high-impact and resistance intervention [ 67 ]. It is interesting that despite the habitual osteogenic stimulus of soccer training, this group of athletes experienced a quantitatively similar increase in section modulus compared to non-weight bearing endurance athletes [ 58 ]. Weight bearing endurance training is not as osteogenic as soccer, and therefore it is likely that WBEA would also improve section modulus following a similar intervention [ 61 ]. Femoral neck section modulus also significantly increased in response to high-impact interventions in inactive premenopausal women [ 64 ] and early pubertal girls [ 65 ]. The only evidence of an association between LEA and estimated bone strength is in terms of failure load estimated under compression. Although resistance to bending at the femoral neck and tibial diaphysis has been shown to improve in response to high-impact interventions, it is not clear whether this would mitigate the effects of LEA or reduce the risk of tibial bone stress injury. There is evidence to suggest that a high-impact intervention may help mitigate the effects of LEA on bone strength; however, further research needs to be conducted before it could be considered a viable tool to protect athletes suffering LEA from bone stress injury. Many of the interventions studied were unidirectional, which is not the optimum loading pattern for osteogenic stimulus [ 23 ], and have only been tested in non-athletes. If WBEA have stronger bones than non-athletes, their response to high-impact interventions may differ [ 55 ]. The only data regarding the effects of a high-impact intervention during LEA are from cross-sectional studies or overweight and postmenopausal populations. Also, to address these issues, future research should aim to test the effects of a multi-directional low repetition high-impact intervention during LEA on bone mineral density, geometry and estimated strength using a longitudinal or controlled design in male and female WBEA. The use of bone re modeling markers to monitor the effects of bone treatment has been supported by the International Osteoporosis Foundation as they respond more rapidly than structural measures such as BMD [ 77 ]. To investigate a cause and effect relationship between LEA and bone health, research has sought to control energy availability and measure the acute change in systemic markers of bone re modeling. Significant reductions in serum pro-peptide of type 1 collagen P1NP; a marker of bone formation were shown in the restricted condition only [ 78 ]. Energy availability per se was not quantified and no measures were taken to prevent the reduction in micronutrient availability associated with energy restriction, which will have influenced the data. These studies by Papageorgiou et al. Findings suggest that both males and females may be affected but females were more sensitive. However, energy availability was not reported and differences in micronutrient availability between conditions may have confounded the data. Papageorgiou et al. Many different re modeling markers exist and heterogeneity between some of the studies makes direct comparisons difficult; however, the findings are consistent with those based on P1NP and β-CTX, which are the recommended international reference markers [ 77 ]. These findings show that LEA suppresses markers of bone formation within five days in active females and male WBEA. Females appear to respond with greater sensitivity than males, which suggests that markers of bone formation may also be suppressed in female WBEA in response to LEA, at least to a similar extent as in male WBEA. In male WBEA, it is possible that markers of bone resorption may only increase if LEA persists for longer than five days. Conversely, markers of bone resorption are increased in response to LEA in active females within five days, but only following LEA of greater duration or severity than is required to suppress markers of bone formation. This implies bone formation is more acutely affected by LEA than bone resorption which may lead to net bone breakdown. Due to a lack of evidence, it remains unclear whether the effect on bone resorption is the same in female athletes versus physically active women. Furthermore, in all studies that restrict energy availability, the availability of at least one of the macronutrients carbohydrate, protein or fat is inevitably reduced in the restricted condition and it has been shown that β-CTX is increased independent of energy availability in situations of reduced carbohydrate availability [ 81 ]. The contribution of reduced macronutrient availability to the effects described could not be determined. The relationships between acute changes in markers of bone formation and resorption in response to LEA and long-term bone health are unclear. Sustained and localized acceleration of bone remodeling may play a role in the pathogenesis of bone stress injury in athletes [ 82 , 83 ]. However, bone re modeling markers are typically measured systemically and, therefore, any change in the balance of such markers does not necessarily reflect a change in bone remodeling at a specific site. Accordingly, prospective research has shown no significant differences in bone re modeling markers between athletes and military recruits who suffered a stress fracture and those who did not [ 84 , 85 ]. It is commonly reported that a reduction in bone resorption leads to long-term bone accrual; however, in an individual bone remodeling unit, resorptive osteoclast cells initiate the remodeling cycle and precede bone formation [ 86 ]. Thus, suppression of resorption might actually inhibit adaptation [ 87 ]. Although the acute effects of LEA on bone re modeling markers cannot yet be interpreted in terms of long-term bone health, it is reasonable to assume that preventing the acute effects from occurring would be beneficial given that evidence suggests the long-term effect of LEA is detrimental to bone structure, strength and stress injury risk. However, the bone re modeling response to repeated days of high-impact exercise is poorly understood. The existing data show either no change or a reduction in markers of bone formation and resorption [ 88 , 89 ], and none of the previous research has simultaneously manipulated energy availability. Also, it is not clear to what degree these findings are due to the interventions per se, or merely a result of biological variation due to poor standardization [ 86 ]. One study was conducted in a cohort of hospitalized anorexia nervosa patients, which provides little information regarding whether high-impact exercise might prevent the acute effects of LEA in active populations [ 89 ]. Furthermore, the markers measured in response to short-term exercise interventions are often different from those measured in response to short-term LEA, which limits direct comparisons. Using prolonged moderate impact running exercise, Papageorgiou et al. There was no effect on β-CTX in any condition [ 79 ]. It was suggested that daily moderate impact exercise might have prevented the acute effects of LEA on bone formation in active individuals. However, it is not known whether this effect persists over a longer period or whether such effects translate to WBEA who are more accustomed to the mechanical loading associated with daily prolonged running. As discussed, the effects of LEA on bone re modeling markers were more marked after five days than after three, but the independent effect of impact exercise has not been tested beyond a period of three days. Future research could use a similar design to that used by Papageorgiou et al. Findings may be used to improve evidence-informed practice, and inform longitudinal studies designed to investigate the effect of such interventions on long-term bone health and stress injury in athletes at risk of LEA. WBEA are frequently exposed to episodes of LEA in association with the demands of their sport. In female athletes, including WBEA, LEA is identified as a causative factor in FHA which, in turn, is associated with impaired bone health, including lower BMD, bone mineral content, and trabecular volumetric BMD, thinner cortices, and reduced bone strength. These impairments have been observed at load bearing sites, such as the proximal femur and tibia, and may increase the risk of bone stress injury as well as osteoporotic fracture. Not until recently was the existence of LEA associated bone loss acknowledged in men and, therefore, there are scarce data in male WBEA. High-impact exercise can be highly osteogenic with a low energy cost. Low repetition high-impact interventions have been shown to increase BMD, cortical thickness and estimated strength and preliminary evidence suggests that some of these effects may occur despite LEA. Such interventions may help attenuate bone change in WBEA and reduce the risk of bone stress injury in those at risk of LEA. Research examining the short-term effects of LEA in active men and women suggests that circulating markers of bone formation may be suppressed and circulating markers of bone resorption may be increased within five days, with women possibly responding to LEA with greater sensitivity. Prolonged moderate impact exercise may help mitigate the effects of short-term LEA; however, it is currently unclear whether this would be of benefit to long-term bone health. Given that bone health in WBEA can become compromised due to LEA, investigation of methods which may protect bone health in the face of LEA is of clinical importance. Lean body mass and fat-free mass are different measures of body composition that have been used interchangeably to normalise energy availability. As this is unlikely to have a major effect in the context of the current review, lean body mass has been reported throughout. Mountjoy M, Sundgot-Borgen J, Burke L, Carter S, Constantini N, Lebrun C, et al. The IOC consensus statement: beyond the Female Athlete Triad-Relative Energy Deficiency in Sport RED-S. Br J Sports Med. Article Google Scholar. Burke LM, Kiens B, Ivy JL. Carbohydrates and fat for training and recovery. J Sports Sci. Article PubMed Google Scholar. Loucks AB, Kiens B, Wright HH. Energy availability in athletes. De Souza MJ, Nattiv A, Joy E, Misra M, Williams NI, Mallinson RJ, et al. M Br J Sports Med. Heikura IA, Uusitalo ALT, Stellingwerff T, Bergland D, Mero AA, Burke LM. Low energy availability is difficult to assess but outcomes have large impact on bone injury rates in elite distance athletes. Int J Sport Nutr Exerc Metab. Article CAS Google Scholar. Loucks AB, Thuma JR. Luteinizing hormone pulsatility is disrupted at a threshold of energy availability in regularly menstruating women. J Clin Endocrinol Metab. Koehler K, Hoerner NR, Gibbs JC, Zinner C, Braun H, De Souza MJ, et al. Low energy availability in exercising men is associated with reduced leptin and insulin but not with changes in other metabolic hormones. Elliott-Sale KJ, Tenforde AS, Parziale AL, Holtzman B, Ackerman KE. Endocrine effects of relative energy deficiency in sport. Int J Sport Nutr Exerc Metab [Internet]. Chen XX, Yang T. Roles of leptin in bone metabolism and bone diseases. J Bone Miner Metab. Article CAS PubMed Google Scholar. Papageorgiou M, Elliott-Sale KJ, Parsons A, Tang JCY, Greeves JP, Fraser WD, et al. Effects of reduced energy availability on bone metabolism in women and men. Tenforde AS, Nattiv A, Barrack M, Kraus E, Kim B, Kussman A, et al. Distribution of bone stress injuries in elite male and female collegiate runners. Med Sci Sport Exerc [Internet]. Bennell KL, Malcolm SA, Thomas SA, Wark JD, Brukner PD. The incidence and distribution of stress fractures in competitive track and field athletes: a twelve-month prospective study. Am J Sports Med. Kelsey JL, Bachrach LK, Procter-Gray E, Nieves J, Greendale GA, Sowers M, et al. Risk factors for stress fracture among young female cross-country runners. Duckham RL, Brooke-Wavell K, Summers GD, Cameron N, Peirce N. Stress fracture injury in female endurance athletes in the United Kingdom: a month prospective study. Scand J Med Sci Sports. Barrack MT, Gibbs JC, De Souza MJ, Williams NI, Nichols JF, Rauh MJ, et al. Higher incidence of bone stress injuries with increasing Female Athlete Triad-related risk factors. Kraus E, Tenforde AS, Nattiv A, Sainani KL, Kussman A, Deakins-Roche M, et al. Bone stress injuries in male distance runners: higher modified Female Athlete Triad cumulative risk assessment scores predict increased rates of injury. Williams NI, Helmreich DL, Parfitt DB, Caston-Balderrama A, Cameron JL. Evidence for a causal role of low energy availability in the induction of menstrual cycle disturbances during strenuous exercise training. Nattiv A, Loucks AB, Manore MM, Sanborn CF, Sundgot-Borgen J, Warren MP. American College of Sports Medicine position. stand The Female Athlete Triad. Med Sci Sports Exerc. Melin A, Tornberg ÅB, Skouby S, Møller SS, Sundgot-Borgen J, Faber J, et al. Energy availability and the Female Athlete Triad in elite endurance athletes. Dusek T. Influence of high intensity training on menstrual cycle disorders in athletes. Croat Med J. CAS PubMed Google Scholar. Mitchell DM, Tuck P, Ackerman KE, Cano Sokoloff N, Woolley R, Slattery M, et al. Altered trabecular bone morphology in adolescent and young adult athletes with menstrual dysfunction. Ackerman KE, Cano Sokoloff N, De Nardo Maffazioli G, Clarke HM, Lee H, Misra M. Fractures in relation to menstrual status and bone parameters in young athletes. Hart NH, Nimphius S, Rantalainen T, Ireland A, Siafarikas A, Newton RU. Mechanical basis of bone strength: influence of bone material, bone structure and muscle action. J Musculoskelet Neuronal Interact. CAS PubMed PubMed Central Google Scholar. Kato T, Terashima T, Yamashita T, Hatanaka Y, Honda A, Umemura Y. Effect of low-repetition jump training on bone mineral density in young women. J Appl Physiol. Bennell KL, Malcolm SA, Thomas SA, Reid SJ, Brukner PD, Ebeling PR, et al. Risk factors for stress fractures in track and field athletes. Nattiv A. Stress fractures and bone health in track and field athletes. J Sci Med Sport. Nattiv A, Kennedy G, Barrack MT, Abdelkerim A, Goolsby MA, Arends JC, et al. Correlation of MRI grading of bone stress injuries with clinical risk factors and return to play. Article PubMed PubMed Central Google Scholar. Tenforde AS, Kraus E, Fredericson M. Bone stress injuries in runners. Phys Med Rehabil Clin N Am. Singhal V, Reyes KC, Pfister B, Ackerman K, Slattery M, Cooper K, et al. Bone accrual in oligo-amenorrheic athletes, eumenorrheic athletes and non-athletes. Ackerman KE, Putman M, Guereca G, Taylor AP, Pierce L, Herzog DB, et al. Cortical microstructure and estimated bone strength in young amenorrheic athletes, eumenorrheic athletes and non-athletes. Pollock N, Grogan C, Perry M, Pedlar C, Cooke K, Morrissey D, et al. Bone-mineral density and other features of the Female Athlete Triad in elite endurance runners: a longitudinal and cross-sectional observational study. Baxter-Jones AD, Faulkner RA, Forwood MR, Mirwald RL, Bailey DA. Bone mineral accrual from 8 to 30 years of age: an estimation of peak bone mass. J Bone Miner Res. Burke LM, Lundy B, Fahrenholtz IL, Melin AK. Pitfalls of conducting and interpreting estimates of energy availability in free-living athletes. Duckham RL, Peirce N, Bailey CA, Summers G, Cameron N, Brooke-Wavell K. Bone geometry according to menstrual function in female endurance athletes. Calcif Tissue Int. Ackerman KE, Nazem T, Chapko D, Russell M, Mendes N, Taylor AP, et al. Bone microarchitecture is impaired in adolescent amenorrheic athletes compared with eumenorrheic athletes and nonathletic controls. J Clin Endocrinol Metab [Internet]. Piasecki J, Ireland A, Piasecki M, Cameron J, McPhee JS, Degens H. The strength of weight-bearing bones is similar in amenorrheic and eumenorrheic elite long-distance runners. Lieberman JL, De Souza MJ, Wagstaff DA, Williams NI. Menstrual disruption with exercise is not linked to an energy availability threshold. Med Sci Sport Exerc. De Souza MJ, Koltun KJ, Williams NI. The role of energy availability in reproductive function in the Female Athlete Triad and extension of its effects to men: an initial working model of a similar syndrome in male athletes. Sport Med. Hackney AC. Hypogonadism in exercising males: dysfunction or adaptive-regulatory adjustment? Front Endocrinol Lausanne. Logue DM, Madigan SM, Melin A, Delahunt E, Heinen M, Donnell S-JM, et al. Low energy availability in athletes an updated narrative review of prevalence, risk, within-day energy balance, knowledge, and impact on sports performance. Nutrients [Internet]. Tenforde AS, Barrack MT, Nattiv A, Fredericson M. Parallels with the Female Athlete Triad in male athletes. Sports Med. Tenforde AS, Fredericson M, Sayres LC, Cutti P, Sainani KL. Identifying sex-specific risk factors for low bone mineral density in adolescent runners. Mallinson RJ, Southmayd EA, De Souza MJ. Duckham RL, Bialo SR, Machan J, Kriz P, Gordon CM. A case-control pilot study of stress fracture in adolescent girls: the discriminative ability of two imaging technologies to classify at-risk athletes. Osteoporos Int. Schnackenburg KE, Macdonald HM, Ferber R, Wiley JP, Boyd SK. Bone quality and muscle strength in female athletes with lower limb stress fractures. Southmayd EA, Mallinson RJ, Williams NI, Mallinson DJ, De Souza MJ. Unique effects of energy versus estrogen deficiency on multiple components of bone strength in exercising women. Popp KL, Frye AC, Stovitz SD, Hughes JM. Bone geometry and lower extremity bone stress injuries in male runners. Ackerman KE, Pierce L, Guereca G, Slattery M, Lee H, Goldstein M, et al. Hip structural analysis in adolescent and young adult oligoamenorrheic and eumenorrheic athletes and nonathletes. Beck T, Ruff C, Shaffer R, Betsinger K, Trone D, Brodine S. Stress fracture in military recruits: gender differences in muscle and bone susceptibility factors. Ruffing JA, Nieves JW, Zion M, Tendy S, Garrett P, Lindsay R, et al. The influence of lifestyle, menstrual function and oral contraceptive use on bone mass and size in female military cadets. Nutr Metab Lond. Pistoia W, van Rietbergen B, Lochmüller E-M, Lill C, Eckstein F, Rüegsegger P. Estimation of distal radius failure load with micro-finite element analysis models based on three-dimensional peripheral quantitative computed tomography images. Sale C, Elliott-Sale KJ. Nutrition and athlete bone health. De Souza MJ, West SL, Jamal SA, Hawker GA, Gundberg CM, Williams NI. The presence of both an energy deficiency and estrogen deficiency exacerbate alterations of bone metabolism in exercising women. McGrath C, Sankaran JS, Misaghian-Xanthos N, Sen B, Xie Z, Styner MA, et al. Exercise degrades bone in caloric restriction, despite suppression of marrow adipose tissue MAT. Frost HM. Anat Rec. Burr DB, Robling AG, Turner CH. Effects of biomechanical stress on bones in animals. Rubin CT, Lanyon LE. Regulation of bone formation by applied dynamic loads. J Bone Joint Surg Am. Vlachopoulos D, Barker AR, Ubago-Guisado E, Williams CA, Gracia-Marco L. A 9-month jumping intervention to improve bone geometry in adolescent male athletes. The effect of a high-impact jumping intervention on bone mass, bone stiffness and fitness parameters in adolescent athletes. Arch Osteoporos. Hinton PS, Nigh P, Thyfault J. Effectiveness of resistance training or jumping-exercise to increase bone mineral density in men with low bone mass: a month randomized, clinical trial. Fredericson M, Chew K, Ngo J, Cleek T, Kiratli J, Cobb K. Regional bone mineral density in male athletes: a comparison of soccer players, runners and controls. One of the potential contributors to this might be an increase in bone resorption mediated by the activation of parathyroid hormone due to reductions in serum calcium levels, which, in turn, occur as the result of dermal calcium losses [ 81 ]. It is likely that the level of dermal calcium loss required to cause a decline in serum calcium concentrations, which is sufficient to activate parathyroid hormone secretion and thus bone demineralisation, would only occur during prolonged hard exercise. Given that calcium plays an important role in many cellular processes that occur while exercising, the body vigorously defends serum calcium concentrations, predominantly by the demineralisation of bone, which, in turn could lead to a reduction in bone mass over time. As such, Barry et al. Barry et al. Twenty male endurance athletes completed a km cycling time trial on three occasions having consumed either 1 mg of calcium 20 min before exercise and a placebo during exercise; 2 a placebo before exercise and mg of calcium every 15 min during exercise; or 3 a placebo before and during exercise. The results showed that when mg of calcium was ingested as a single bolus prior to exercise, there was an attenuated parathyroid hormone response to the subsequent exercise bout. There was a smaller attenuation of the parathyroid hormone response when calcium was supplemented during exercise, and this did not reach statistical significance. This latter possibility has not been explored and future research is required. In line with this, there is also the possibility that the challenge to fluid and sodium homeostasis that would occur under these circumstances might influence bone metabolism and health. This, to our knowledge, has not been directly or well-studied in relation to the athlete, but there is some suggestion from the osteoporosis focussed literature suggesting that bone might be negatively affected by hyponatraemia. Verbalis et al. The same paper also reported on a cross-sectional analysis of human adults from the Third National Health and Nutrition Examination Survey, showing that mild hyponatraemia was associated with significantly increased odds of osteoporosis, in line with the rodent data presented. This might be explained by novel sodium signalling mechanisms in osteoclasts resulting in the release of sodium from bone stores during prolonged hyponatraemia [ 84 ]. Nutrient ingestion around acute exercise can alter the bone resorption marker response to that exercise bout. Many athletes exercise in the morning after an overnight fast, which has the potential to promote an increase in bone turnover. Scott et al. As anticipated, the ingestion of food reduced pre-exercise bone resorption as measured by β-CTX , but, contrary to what was proposed, the bone resorption response to exercise was greater in the fed condition than in the fasted condition and, over time, there was no difference in the response between fasting and feeding. As such, it seemed that the mechanical loading induced by exercise might have provided a more powerful stimulus than that of pre-exercise feeding. In line with this theory, Sale et al. Carbohydrate feeding attenuated bone resorption β-CTX and formation P1NP in the hours but not days following exercise, indicating an acute effect of carbohydrate feeding on bone turnover. The total amount of glucose ingested was Given the fact that the post-exercise period might provide a longer timeframe and a greater scope for intervention, Townsend et al. There were three trials conducted in this study: 1 placebo: ingested immediately and 2 h post-exercise; 2 immediate feeding: carbohydrate plus protein 1. When carbohydrate plus protein was ingested immediately post-exercise, there was a suppression of the exercise-induced bone resorption β-CTX response when compared to the control trial, along with a smaller increase in the bone formation P1NP response 3—4 h post-exercise. It would seem clear that feeding around exercise can moderate the bone metabolic response to that exercise bout, with the post-exercise period being perhaps the most useful timeframe for intervention. Longer-term studies are therefore required to determine whether or not these shorter-term or acute responses to feeding around exercise are positive for bone health. The studies in this area have largely been conducted in men, and it would be of interest to determine whether the same effects are seen in exercising women. Bone health is an important issue for some athletes, particularly those who are at a greater risk of low or lower BMD. These athletes should develop strategies to take care of their bones, particularly during adolescence and early adulthood, even at the expense of their training and performance, given that trying to overcome an already low bone mass in later life is extremely difficult. Taking care of their diet and nutrition might help athletes to better protect their bones against the demands of their sport. Dietary advice for athletes in this regard should remain in line with the advice given to the general population, with some consideration given to where there would be a need for higher intakes to match the needs of the sport and to optimise function, although there are several specific challenges that certain athletes might face over and above those faced by the general population. In this review, we have attempted to acknowledge some of these potential issues and highlight the information that is currently available to support these views. There is, however, a dearth of information relating to the effects particularly the longer-term effects of different dietary and nutritional practices on bone health in athletes, and significant research effort is required on this topic in the future. There is still a requirement to clearly define which types of athlete are and which types of athlete are not at risk of longer-term bone health issues, such as osteopenia and osteoporosis. Further research is needed to determine the wider implications of reduced energy availability, beyond bone, as suggested by the RED-S syndrome; currently these are not well researched. It remains to be clearly established whether there is or is not a male athlete triad and whether the bone health implications of reduced energy availability are seen at the same level as in females or whether males are a little more resistant to the effects of low energy availability. Further research is required into the periodisation of low energy availabilities in endurance athletes, such that they can benefit from the positive effects of calorie restriction on the endurance phenotype but without putting their bone health at risk. More work is required in athletes to determine the effects of nutrient availability particularly of carbohydrate separately from energy availability on bone health. The amounts of calcium lost during training in endurance and ultra-endurance athletes are still not well known, nor is the amount of calcium lost during more passive sweating, particularly in hot environments, such as might be performed by weight-making athletes. No research has been conducted in athletes to determine whether or not there is an effect of sweat sodium loss on bone. Longer-term studies are needed to determine whether or not the shorter-term or acute responses of bone metabolism to feeding are positive for bone health. These studies should also seek to determine whether feeding should be periodised around hard training blocks rather than constant so as not to reduce the potential adaptation of the bone to exercise training. Santos L, Elliott-Sale KJ, Sale C. Exercise and bone health across the lifespan. CAS PubMed PubMed Central Google Scholar. Dobbs MB, Buckwalter J, Saltzman C. Osteoporosis: the increasing role of the orthopaedist. Iowa Orthop J. Johnell O, Kanis J. Epidemiology of osteoporotic fractures. Osteoporos Int. PubMed Google Scholar. World Health Organization. Assessment of fracture risk and its application to screening for postmenopausal osteoporosis. Technical Report Series, Hernlund E, Svedbom A, Ivergård M, Compston J, Cooper C, Stenmark J, et al. Osteoporosis in the European Union: medical management, epidemiology and economic burden. A report prepared in collaboration with the International Osteoporosis Foundation IOF and the European Federation of Pharmaceutical Industry Associations EFPIA. Arch Osteoporos. National Institute for Health and Clinical Excellence. Osteoporosis fragility fracture risk—Costing report. Recker RR, Davies KM, Hinders SM, Heaney RP, Stegman MR, Kimmel DB. Bone gain in young adult women. CAS PubMed Google Scholar. Henry YM, Fatayerji D, Eastell R. Attainment of peak bone mass at the lumbar spine, femoral neck and radius in men and women: relative contributions of bone size and volumetric bone mineral density. Ranson CA, Burnett AF, Kerslake RW. Injuries to the lower back in elite fast bowlers: acute stress changes on MRI predict stress fracture. J Bone Jt Surg. CAS Google Scholar. Scofield KL, Hecht S. Bone health in endurance athletes: runners, cyclists and swimmers. Curr Sports Med Rep. Dolan E, McGoldrick A, Davenport C, Kelleher G, Byrne B, Tormey W, et al. An altered hormonal profile and elevated rate of bone loss are associated with low bone mass in professional horse-racing jockeys. J Bone Miner Metab. Wilson G, Hill J, Sale C, Morton JP, Close GL. Elite male flat jockeys display lower bone density and lower resting metabolic rate than their female counterparts: implications for athlete welfare. Appl Physiol Nutr Metab. Amorim T, Koutedakis Y, Nevill A, Wyon M, Maia J, Machado J, et al. Bone mineral density in vocational and professional ballet dancers. Wewege MA, Ward RE. Bone mineral density in pre-professional female ballet dancers: a systematic review and meta-analysis. J Sci Med Sport. Frost HM. The mechanostat: a proposed pathogenetic mechanism of osteoporoses and the bone mass effects of mechanical and nonmechanical agents. Bone Miner. Clowes JA, Hannon RA, Yap TS, Hoyle NR, Blumsohn A, Eastell R. Effect of feeding on bone turnover markers and its impact on biological variability of measurements. Walsh JS, Henriksen DB. Feeding and bone. Arch Biochem Biophys. Babraj JA, Smith K, Cuthbertson DJ, Rickhuss P, Dorling JS, Rennie MJ. Human bone collagen synthesis is a rapid, nutritionally modulated process. J Bone Miner Res. Schlemmer A, Hassager C. Acute fasting diminishes the circadian rhythm of biochemical markers of bone resorption. Eur J Endocrinol. Mitchell PJ, Cooper C, Dawson-Hughes B, Gordon CM, Rizzoli R. Life-course approach to nutrition. Palacios C. The role of nutrients in bone health, from A to Z. Crit Rev Food Sci Nutri. Jugdaohsingh R. Silicon and bone health. J Nutr Health Ageing. Price CT, Langford JR, Liporace FA. Essential nutrients for bone health and a review of their availability in the average North American diet. Open Orthop J. PubMed PubMed Central Google Scholar. Larson-Meyer ED, Woolf K, Burke L. Assessment of nutrient status in athletes and the need for supplementation. Int J Sports Nutr Exerc Metab. Nattiv A, Loucks AB, Manore MM, Sanborn CF, Sundgot-Borgen J, Warren MP, et al. American College of Sports Medicine position stand. The female athlete triad. Med Sci Sports Exerc. Logue D, Madigan SM, Delahunt E, Heinen M, McDonnell SJ, Corish CA. Low energy availability in athletes: a review of prevalence, dietary patterns, physiological health, and sports performance. Sports Med. Heikura IA, Uusitalo ALT, Stellingwerff T, Bergland D, Mero AA, Burke LM. Low energy availability is difficult to assess but outcomes have large impact on bone injury rates in elite distance athletes. Papageorgiou M, Dolan E, Elliott-Sale KJ, Sale C. Reduced energy availability: implications for bone health in physically active populations. Eur J Nutr. Loucks AB, Kiens B, Wright HH. Energy availability in athletes J Sports Sci. Slater J, McLay-Cooke R, Brown R, Black K. Female recreational exercisers at risk for low energy availability. Google Scholar. Torstveit MK, Fahrenholtz IL, Lichtenstein MB, Stenqvist TB, Melin AK. Exercise dependence, eating disorder symptoms and biomarkers of relative energy deficiency in sports RED-S among male endurance athletes. BMJ Open Sport Exerc Med. Ihle R, Loucks AB. Dose-response relationships between energy availability and bone turnover in young exercising women. Vasikaran S, Cooper C, Eastell R, Griesmacher A, Morris HA, Trenti T, et al. Markers of bone turnover for the prediction of fracture risk and monitoring of osteoporosis treatment: a need for international reference standards. Thong FS, McLean C, Graham TE. Plasma leptin in female athletes: relationship with body fat, reproductive, nutritional, and endocrine factors. J Appl Physiol. Papageorgiou M, Elliott-Sale KJ, Parsons A, Tang JCY, Greeves JP, Fraser WD, et al. Effects of reduced energy availability on bone metabolism in women and men. Papageorgiou M, Martin D, Colgan H, Cooper S, Greeves JP, Tang JCY, et al. Bone metabolic responses to low energy availability achieved by diet or exercise in active eumenorrheic women. Prouteau S, Pelle A, Collomp K, Benhamou L, Courteix D. Bone density in elite judoists and effects of weight cycling on bone metabolic balance. Ackerman KE, Nazem T, Chapko D, Russell M, Mendes N, Taylor AP, et al. Bone microarchitecture is impaired in adolescent amenorrheic athletes compared with eumenorrheic athletes and nonathletic controls. J Clin Endocrinol Metab. Ackerman KE, Putman M, Guereca G, Taylor AP, Pierce L, Herzog DB, et al. Cortical microstructure and estimated bone strength in young amenorrheic athletes, eumenorrheic athletes and non-athletes. De Souza MJ, West SL, Jamal SA, Hawker GA, Gundberg CM, Williams NI. The presence of both an energy deficiency and estrogen deficiency exacerbate alterations of bone metabolism in exercising women. Southmayd EA, Mallinson RJ, Williams NI, Mallinson DJ, De Souza MJ. Unique effects of energy versus estrogen deficiency on multiple components of bone strength in exercising women. De Souza MJ, Nattiv A, Joy E, Misra M, Williams NI, Mallinson RJ, et al. Br J Sports Med. Tenforde AS, Barrack MT, Nattiv A, Fredericson M. Parallels with the female athlete triad in male athletes. Mountjoy M, Sundgot-Borgen J, Burke L, Carter S, Constantini N, Lebrun C, et al. The IOC consensus statement: beyond the female athlete triad—relative energy deficiency in sport RED-S. Mountjoy M, Sundgot-Borgen JK, Burke LM, Ackerman KE, Blauwet C, Constantini N, et al. IOC consensus statement on relative energy deficiency in sport RED-S : update. Stellingwerff T. Case study: body composition periodization in an Olympic-level female middle-distance runner over a 9-year career. Petkus DL, Murray-Kolb LE, De Souza MJ. The unexplored crossroads of the female athlete triad and iron deficiency: a narrative review. Noakes T, Volek JS, Phinney SD. Low-carbohydrate diets for athletes: what evidence? Br J Sports Nutr. Chang CK, Borer K, Lin PJ. Low-carbohydrate-high-fat diet: can it help exercise performance? J Hum Kinet. Bjarnason NH, Henriksen EE, Alexandersen P, Christgau S, Henriksen DB, Christiansen C. Mechanism of circadian variation in bone resorption. de Sousa MV, Pereira RM, Fukui R, Caparbo VF, da Silva ME. Carbohydrate beverages attenuate bone resorption markers in elite runners. Sale C, Varley I, Jones TW, James RM, Tang JC, Fraser WD, et al. Effect of carbohydrate feeding on the bone metabolic response to running. Bielohuby M, Matsuura M, Herbach N, Kienzle E, Slawik M, Hoeflich A, et al. Short-term exposure to low-carbohydrate, high-fat diets induces low bone mineral density and reduces bone formation in rats. Carter JD, Vasey FB, Valeriano J. The effect of a low-carbohydrate diet on bone turnover. Morton RW, Murphy KT, McKellar SR, Schoenfeld BJ, Henselmans M, Helms E, et al. A systematic review, meta-analysis and meta-regression of the effect of protein supplementation on resistance training-induced gains in muscle mass and strength in healthy adults. Kraut J, Coburn J. Bone, acid and osteoporosis. N Engl J Med. Barzel U, Massey L. Excess dietary protein can adversely effect bone. J Nutr. Dolan E, Sale C. Protein and bone health across the lifespan. Proc Nutr Soc. Fenton T, Eliasziw M, Lyon A, Tough SC, Hanley DA. Meta-analysis of the quantity of calcium excretion associated with the net acid excretion of the modern diet under the acid ash diet hypothesis. Am J Clin Nutr. Macdonald HM, New SA, Fraser WD, Campbell MK, Reid DM. Low dietary potassium intakes and high dietary estimates of net endogenous acid production are associated with low bone mineral density in premenopausal women and increased markers of bone resorption in postmenopausal women. The impact of dietary protein on calcium absorption and kinetic measures of bone turnover in women. Heaney R. Bone Health. Zimmerman E, Busse B, Ritchie R. The fracture mechanics of human bone: influence of disease and treatment. Bonekey Rep. Do multi-ingredient protein supplements augment resistance training-induced gains in skeletal muscle mass and strength? A systematic review and meta-analysis of 35 trials. Article PubMed Google Scholar. Kohrt WM, Barry DW, Schwartz RS. Muscle forces or gravity: what predominates mechanical loading on bone? Rizzoli R, Biver E, Bonjour JP, Coxam V, Goltzman D, Kanis JA, et al. Benefits and safety of dietary protein for bone health—an expert consensus paper endorsed by the European Society for Clinical and Economical Aspects of Osteoporosis, Osteoarthritis, and Musculoskeletal Diseases and by the International Osteoporosis Foundation. Owens DJ, Fraser WD, Close GL. Vitamin D and the athlete: emerging insights. Eur J Sport Sci. Pearce SH, Cheetham TD. Diagnosis and management of vitamin D deficiency. Scientific Advisory Committee on Nutrition. Vitamin D and Health. Accessed 17 Oct The Institute of Medicine. Dietary Guidelines for Americans Holick MF. Vitamin D deficiency. Angeline ME, Gee AO, Shindle M, Warren RF, Rodeo SA. The effects of vitamin D deficiency in athletes. Am J Sports Med. Cannell JJ, Hollis BW, Sorenson MB, Taft TN, Anderson JJ. Athletic performance and vitamin D. Miller JR, Dunn KW, Ciliberti LJ, Patel RD, Swanson BA. Association of vitamin D with stress fractures: a retrospective cohort study. J Foot Ankle Surg. Maroon JC, Mathyssek CM, Bost JW, Amos A, Winkelman R, Yates AP, et al. Vitamin D profile in National Football League players. Lappe J, Cullen D, Haynatzki G, Recker R, Ahlf R, Thompson K. Calcium and vitamin D supplementation decreases incidence of stress fractures in female navy recruits. Nieves JW, Melsop K, Curtis M, Kelsey JL, Bachrach LK, Greendale G, et al. Nutritional factors that influence change in bone density and stress fracture risk among young female cross-country runners. Institute of Medicine. Dietary reference intakes for calcium and vitamin D: Institute of Medicine of the National Academies, Rector RS, Rogers R, Ruebel M, Hinton PS. Participation in road cycling vs running is associated with lower bone mineral density in men. Tenforde AS, Carlson JL, Sainani KL, Chang AO, Kim JH, Golden NH, et al. Sport and triad risk factors influence bone mineral density in collegiate athletes. Barry DW, Hansen KC, van Pelt RE, Witten M, Wolfe P, Kohrt WM. Acute calcium ingestion attenuates exercise-induced disruption of calcium homeostasis. Haakonssen EC, Ross ML, Knight EJ, Cato LE, Nana A, Wluka AE, et al. The effects of a calcium-rich pre-exercise meal on biomarkers of calcium homeostasis in competitive female cyclists: a randomised crossover trial. PLoS One. Verbalis JG, Barsony J, Sugimura Y, Tian Y, Adams DJ, Carter EA, et al. Hyponatremia-induced osteoporosis. Barsony J, Sugimura Y, Verbalis JG. Osteoclast response to low extracellular sodium and the mechanism of hyponatremia-induced bone loss. J Biol Chem. Scott JP, Sale C, Greeves JP, Casey A, Dutton J, Fraser WD. Effect of fasting versus feeding on the bone metabolic response to running. |
Strength training builds more than muscles - Harvard Health | Cooke, … Julie M. Although the relevance of some of these markers of bone metabolism was questioned they would not be considered the optimal markers of bone resorption and formation to use today [ 33 ] , this paper has been instrumental in raising the awareness of potential problems for the bone when energy availability is low. CAS PubMed PubMed Central Google Scholar Haakonssen EC, Ross ML, Knight EJ, Cato LE, Nana A, Wluka AE, et al. Low energy availability is difficult to assess but outcomes have large impact on bone injury rates in elite distance athletes. Compr Physiol. Sale, C. |
Media Contact | However, recent data Bonee that athletes who specialize at healh young age Boone at a greater risk of an overuse injury and healt Bone health for power athletes likely to progress xthletes Bone health for power athletes levels of Bonee. Article CAS Bone health for power athletes Scholar Frost HM. Gut health and food cravings present Gut health supplements suggest that lifetime competitive activity has a positive effect on BMD and BMC in masters athletes. Energy availability can be described as the amount of ingested energy remaining to support basic bodily functions and physiological processes, including growth, immune function, locomotion, and thermoregulation, once the energy needed for exercise has been utilised [ 25 ]. Article PubMed Google Scholar Nguyen TV, Center JR, Eisman JA. Thus, masters athletes should have greater bone mineral density than aged-matched non-athletes and inactive peers [ 1314 ]. |
Main Content | The Athlehes causes of the powwr decline in bone Bkne density are reduced supply and absorption of calcium, hormonal changes, Curcumin Supplements decreasing atthletes of physical activity [ 2 ]. Effectiveness Bone health for power athletes resistance poeer or jumping-exercise to increase bone mineral density in men with low bone mass: a month randomized, clinical trial. Osteoporosis International. In cases with spinal osteoporosis, avoiding flexion and twisting activities is encouraged Briggs et al, The relationship between body composition and bone mineral content: threshold effects in a racially and ethnically diverse group of men. Google Scholar Ma D, Wu L, He Z. |
Ja Sie der talentvolle Mensch
Ich denke, dass Sie sich irren. Schreiben Sie mir in PM, wir werden reden.
Sie lassen den Fehler zu. Es ich kann beweisen. Schreiben Sie mir in PM, wir werden reden.
Ich entschuldige mich, aber meiner Meinung nach sind Sie nicht recht. Es ich kann beweisen. Schreiben Sie mir in PM.