Chitosan for bone health -
However, the antibacterial properties of SCS have rarely been reported Green et al. Adding SCS to the BMP2-loaded calcium-deficient HA scaffold can increase the cumulative release of BMP2 compared with the scaffold without SCS.
This composite scaffold promoted the repair of skull defect in a rat model Zhao et al. In another study, 2-N-SCS, 6-O-SCS, and 2-N, 6-O-sulfated chitosan 26SCS were successfully synthesized.
Among them, 26SCS is the most suitable enhancer for BMP2 with a sulfur content of Low-dose 26SCS 0. These effects may be related to the fact that 26SCS can increase the expression levels of noggin NOG mRNA. Nevertheless, SCS alone does not promote the osteogenic differentiation of C2C12 myoblast cells.
This finding indicated that SCS should work together with BMP2 to indirectly affect osteogenic differentiation Zhou et al. The preparation of a photopolymerisable hydrogel incorporating recombinant human BMP2 rhBMP2 -loaded 26SCS-based nanoparticles can promote the attachment and osteogenic differentiation of human MSC in vitro.
New bone formation was found in a rabbit model of the radial defect Cao et al. Therefore, we conclude that SCS alone cannot be used as a bone-forming material.
To achieve this purpose, it needs to be combined with osteogenic biomaterials, such as BMP2. Additionally, the combination of SCS with metal ions or antibacterial substances can be explored to improve the antibacterial properties and serve as a synergistic factor in the regeneration of local infected bone defects.
Compared with CS, ionic conductivity, and swelling index are both improved in PCS. Although the crystallinity was reduced, the tensile strength remained similar to that of CS.
Unlike CS, PCS has a significantly rough surface Jayakumar et al. PCS is mainly produced through two approaches. The first approach involves the reaction between the CS hydroxyl group and phosphorus pentoxide group in the presence of methanesulfonic acid, which can be used as a protective agent for the CS amino group Jayakumar et al.
The second approach involves the reaction of CS hydroxyl functional groups with phosphoric acid in the presence of urea Jayakumar et al.
Owing to the presence of the phosphate group, PCS has metal chelating ability. It can be combined with calcium phosphate crystal particles, thus improving the mechanical properties of PCS and promoting bone properties. Li et al. Studies on the osteogenic effect of PCS have shown that it modulates the expression levels of osteoclastogenic factors, nuclear factor κB ligand-receptor activator RANKL , and osteoprotegerin OPG protein.
Moreover, it may inhibit osteoclast differentiation by upregulating the expression ratio of OPG and RANKL in human primary osteoblasts Tang et al.
These scaffolds promoted bone healing in an ulna defect model in vivo Chen et al. Collectively, these properties ensure that PCS can be used as a potential biomaterial in the treatment of infected bone defects. This part describes the rarely modified CS; many functions have not been confirmed thus far, and the osteogenesis and antibacterial properties require further investigation.
Thiolated chitosan TCS can be obtained by adding the thiol group to the primary amino group of CS, which can improve some properties of CS e. Succinylation of CS can effectively augment water solubility and biocompatibility and yield new functional groups.
The succinylated CS hydrogel can controllably release drugs under the influence of pH. Through combination with bone graft material, this hydrogel can increase the rates of cell growth and bone differentiation.
Hydroxypropyl CS and hydroxybutyl CS are two forms of hydroxyalkyl CS. Hydroxyalkyl CS was obtained by the substitution reaction of CS and epoxide on amino or hydroxyl groups; however, it is rarely used in the treatment of bone defects LogithKumar et al.
aureus within only 30 min. In addition, hydroxybutyl CS can rapidly form a gel, stably exist in vivo , and is injectable Peng et al. The ethylene glycol CS has better mechanical properties and a slower degradation rate than pure CS Huang et al.
The above modified CS is rarely reported. Although its performance has been improved, its synthesis may be more complicated. Further investigation is warranted to improve its performance and render it suitable for the treatment of infected bone defects.
In addition to the chemical modification of CS, mixing CS with other materials e. CS can be used as a carrier for the delivery of antibacterial drugs and osteogenic molecules e. This part summarizes the application of CS-based biomaterials in the treatment of infected bone defects.
The CS hydrogel scaffold has a 3D porous structure, which can simulate the microenvironment of the ECM, promote cell adhesion and proliferation, and allow nutrient and metabolite exchange and cell migration. Moreover, it can encapsulate osteoblasts or growth factors to promote the regeneration of bone tissue Sultankulov et al.
The CS-based thermosensitive hydrogel has recently attracted attention in bone tissue engineering. CS itself is not a thermosensitive polymer, however, through modification, thermosensitivity can be realized. For example its thermal gelation can achieved by adding GP to the CS solution Ji et al.
GP is a natural organic compound that exists in the body and one of the components of the osteogenic medium. It can promote the differentiation of MSC into osteoblasts by extracellular related kinases Wang et al.
This type of hydrogel can exist in a fluid state at room temperature. Following injection into the body, it forms an in-situ stable hydrogel under body temperature conditions Zhou et al.
This property of the CS hydrogel allows it to be injected directly into the bone defect cavity, regardless of the shape and size. Moreover, changing from the original liquid state to the gel state can make it act as a favorable drug carrier.
Nevertheless, it is also characterized by certain shortcomings, such as mechanical stability, and insufficient osteoconductivity.
Some modification methods have been developed to solve this problem. Huang et al. The gel showed good stability, which may be related to the hydrogen bond formed between collagen and CS.
This hydrogel system showed enhanced mechanical properties and ability to induce osteogenesis. To further improve the osteogenic properties of the CS hydrogel, Jayash et al. produced an OPG-CS gel for the treatment of critical-sized skull defects in rabbits.
Rabbits in the OPG-CS gel group exhibited more obvious new bone formation at 6 and 12 weeks after treatment compared with those in the CS gel group Jayash et al. aureus both in vitro and in vivo Figure 4 Tao et al.
FIGURE 4. Injectable thermosensitive hydrogel system for the delivery of VCM in the treatment of osteomyelitis Tao et al. A Schematic diagram of the synthesis of antibacterial and osteogenic hydrogels. aureus with different concentrations of VCM; c Agar plate diffusion of different groups at 1 and 15 days.
D a Histopathology and IL6 immunohistochemical analysis of the rabbit tibia; b , c Quantitative analysis of new bone formation and d Quantitative analysis of IL-6 immunohistochemical staining. aureus , Staphylococcus aureus ; VCM, vancomycin; ZOI, zone of inhibition.
In addition, CS can be modified and mixed with other materials to improve its performance. Single-component polymer hydrogels may have poor mechanical properties and insufficient cell adhesion. Sun et al. This dual-network hydrogel has excellent mechanical properties, high crosslinking density, and low swelling degree.
In an in vivo rat model of skull bone defects, it was demonstrated that addition of BMP2 to this hydrogel can significantly augment new bone formation and biocompatibility. In addition, modification of this hydrogel with thiolated halloysites improves adhesion to MC3T3-E1 cells and further promotes proliferation.
This is related to the participation of thiol on the outer surface in the disulfide exchange during the process of cell adhesion, which is mediated by fibronectin and collagen Rosenberg et al.
Traditional methods e. Guanidinylated CS supramolecular hydrogels, which are driven by reversible non-covalent bonds, include ionic interaction and hydrophobic interaction hydrogen bonds, and have been associated with self-healing and injectable properties Zhang et al.
A major goal in the field of orthopedic implant materials is to provide a bio-interactive surface that can prevent bacterial adhesion and enhance biological activity Leedy et al.
The osteoconductivity, biodegradability, antibacterial, drug delivery properties, and flexibility of processing and modification of CS render it a potential coating material for orthopedic implants.
The conventional preparation processes for CS coating include electrophoretic deposition EPD , spin coating, electrostatic spinning, and sol-gel methods Kumari et al. EPD has become one of the most commonly used methods for the preparation of coatings due to its ability to control the coating composition and complex shape, high efficiency, simplicity, and the absence of a need for a crosslinking agent Obregón et al.
CS is also highly positively charged; thus, it is easy to prepare a coating using the cathodic EPD method. The use of CS as a coating material has two main functions. Firstly, it can induce calcium deposition on the surface of the coating, thus improving the mineralization ability and promoting osteogenesis.
Secondly, as a carrier for the delivery of antibiotics, biomaterials, and growth factors, the CS coating has many advantages, such as a controllable drug release rate and a slow degradation rate Frank et al.
Coating CS on titanium alloy scaffold to delivery ciprofloxacin can strongly inhibit S. aureus , and does not affect the osteogenic activity of MG63 osteoblast-like cells in vitro.
Beenken et al. In the absence of CS coating, the concentration of daptomycin eluted from the calcium phosphate particles was rapidly decreased.
In a rabbit model, calcium sulfate pellets with CS coating exerted a better effect on osteomyelitis than those without CS coating. Nancy et al. This double-layer coating has both antibacterial and osteogenic properties, and can sustain the release of vancomycin over 48 h.
In bone tissue engineering, porous bioglass is also commonly used as a carrier of osteogenic or antibacterial drugs.
However, it is often characterized by burst release of drugs, which shortens the duration of its effectiveness Soundrapandian et al. This shortcoming can be overcome by combining CS with bioactive glass, which can effectively prolong the release cycle to prevent the occurrence of osteomyelitis.
Patel et al. Ampicillin was eluted continuously from the CS-bioactive glass nanoparticles coating for 10—11 weeks, confirming its ability for long-term drug delivery.
Besides CS molecules, calcium and silicon ion products eluted from bioactive glass nanoparticles can promote the osteogenic ability of MC3T3-E1 cells. Furthermore, bioactive glass nanoparticles and CS can jointly promote the formation of apatite on the coating surface and enhance the mineralization ability.
Because of their hydrophobicity, most synthetic polymeric materials do not support cell adhesion, proliferation and differentiation.
The surface coating formed with CS can enhance the surface hydrophilicity of the polymer material, thereby increasing the cell adhesion Tong et al.
Due to the presence of CS, the scaffold can promote the adhesion and proliferation of rat BMSC, and upregulate the expression of osteogenesis-related genes e. FIGURE 5. Microspheres are flowing particles typically composed of inorganic or polymer materials.
Generally, microspheres are thought to be excellent cell or drug transport vehicles. Notably, microspheres have a large specific surface area; therefore, modification of their surfaces may promote cell—or drug—substrate contact Park et al.
CS has good biocompatibility and biodegradability. Sintering, coagulation precipitation, and emulsion crosslinking methods are the most widely utilized processes for producing microspheres from CS Huang et al. Using the sintering microsphere technique, Jiang et al.
The mechanical properties of the scaffold were within the range of the human trabecular bone, and the degradation rate was slower than that recorded for the pure PLGA scaffold. Addition of heparin and BMP2 to the scaffold promoted bone formation at the bone defect in the early stage Figure 6.
The acidic products of some polymers e. In contrast, the metabolites of CS are neutral or slightly alkaline, which is beneficial to the adhesion, proliferation, and differentiation of cells Dias et al.
By adjusting the proportions of CS microspheres and calcium phosphate cements, the absorbability can be improved without affecting the overall compressive strength. In rabbit model of femoral defects, at 24 weeks after implantation, the CS microsphere scaffold had been mostly absorbed and a large number of new bones was observed in the transplantation area Meng et al.
This is attributed to the fact that CS in the form of microspheres can promote the degradation of calcium phosphate cements and the formation of new bones.
In addition, CS microspheres prepared by the emulsion crosslinking method showed better compatibility and osteogenesis with BMSC. Moreover, the degree of bone regeneration in vivo was greater than that obtained via the coagulation precipitation method Xu et al.
Therefore, differences in the preparation of CS microspheres will affect cell expression and bone regeneration. In the treatment of infected defects, appropriate preparation methods should be selected to maximize the utilization of CS microspheres.
FIGURE 6. A The X-ray images of the ulna defect a , X-ray b, c, d at 4, 8, and 12 weeks after implantation in the HP-CS-PLGA-BMP2 group. Three-dimensional CT, e—h and i—l at 6 and 12 weeks after implantation in the PLGA, CS-PLGA, HP-CS-PLGA, and HP-CS-PLGA-BMP2 groups, respectively.
BMP2, bone morphogenetic protein 2; CS, chitosan; CT, computed tomography; HP, heparin; PLGA, and poly lactic-co-glycolic acid. As a nanofiber scaffold material, its unique characteristics i. Nanofiber scaffolds can mimic the nanoscale characteristics of the ECM, promote cell adhesion and migration, and enhance metabolism and the transport of nutrients Sofi et al.
As special biomaterials with nanometer size, CS nanofibers scaffolds can be fabricated by electrospinning, self-assembly, thermal separation, ultrasonic treatment, and chemical synthesis Ding et al. Among them, electrospinning is the most commonly used technology for preparing CS nanofibers.
By altering the parameters of electrospinning e. Compared with CS films, CS nanofibers prepared through electrospinning can better promote the adhesion and proliferation of mouse osteoblasts. Moreover, CS nanofibers can stimulate the proliferation and maturation of osteoblasts by inducing the runt-related transcription factor 2 RUNX2 -mediated regulation of OPN, OCN, and ALP gene expression in osteoblasts through the BMP signaling pathway Ho et al.
In a model of femoral defects, implantation of the CS nanofiber scaffold promoted bone healing by stimulating and improving the quantity and quality of trabecular bone formation Ho et al. CS nanofibers can also improve the mechanical properties of composite biomaterial scaffolds.
The fabrication of high-strength nanofiber scaffolds is a major focus in the field of bone defect therapy. The excellent mechanical properties of such scaffolds contribute to maintaining the structural stability of biomaterials in vivo.
CS lacks sufficient mechanical properties; hence, the mechanical strength of nanofibers can be optimized by combining CS with other materials. By simulating the modulus of bone, this composite scaffold can also enhance the differentiation ability of osteoblast precursor cells and ECM deposition.
Therefore, this type of scaffold can be used as a biological template for the formation of new bone Frohbergh et al. A novel type of PRP-incorporated electrospun polyvinyl-alcohol-CS-HA nanofibers exhibited remarkable biological and mechanical properties, similar to those of human tissue.
Furthermore, this composite scaffold improved the ability of osteoblasts for adhesion and proliferation Abazari et al. In addition to physical properties, such as mechanical properties and porosity, composite nanofibers containing CS may offer antibacterial activity.
The use of the copper I —catalyzed azide-alkyne cycloaddition CuAAC reaction to graft polycaprolactone PCL to the CS by the electrospinning method has been reported. The triazole group produced by the CuAAC reaction can interact with lipids on the microbial cell membrane to produce antibacterial properties and enhance the osteoblast activity of MG cells Sedghi et al.
Although electrospinning is one of the most commonly used methods in bone tissue engineering, it is associated with some challenges. For example, the selection of solvents will affect the cytotoxicity of CS nanofibers, and the process of electrospinning is relatively complicated Chahal et al.
The preparation of polycationic CS and polyanionic ulvan nanofibers by the molecular self-assembly method can also promote the proliferation of osteoblasts and maintain the morphology of osteoblasts.
The manufacturing method is simpler than that of the electrospinning method Toskas et al. The side chain groups of CS can also be modified to prepare nanofibers.
Compared with the solvent used for electrospinning CS nanofibers, CMC nanofibers are water-soluble, non-toxic, and do not require the removal of acid salts generated during electrospinning Su et al. The CMC nanofibers with HA can be prepared by the electrospinning method through simple biomimetic mineralization.
CMC nanofibers have more mineral deposits than CS nanofibers at 16 h after mineralization. This observation is mainly attributed to the fact that carboxymethyl groups provide more nucleation sites, which is consistent with the findings described above. CMC nanofibers can effectively promote the differentiation of mouse BMSC in vitro and augment osteogenesis in rat calvarial bone defects in vivo Figure 7 Zhao et al.
FIGURE 7. CMC nanofibers have a biomimetic mineralization function in vitro and in vivo Zhao et al. Infected bone defects continue to pose a challenge in the field of orthopedics. CS is a bioactive material commonly used in bone tissue engineering. CS kills bacteria through the combination of positive and negative charge.
Moreover, CS promotes the proliferation of osteoblasts by increasing the expression of genes related to calcium binding and mineralization, such as ALP, OPN, and OCN. Also, combination with inorganic and organic molecules e. In addition, these properties of CS can be enhanced by side chain modification, yielding QCS, CMC, SCS, and PCS.
In addition, carboxylation, sulfation, and phosphorylation also promote the antibacterial and osteogenic properties of CS. At present, CS can be applied to the treatment of infected defects in many forms, including hydrogels, coatings, microspheres, and nanofibers, all of which have achieved good therapeutic effects.
Although there have been significant advances in the research on the treatment of infectious bone defects with CS, there are still some deficiencies that need to be implemented to promote its extensive clinical application.
First of all, the hydrophobicity of CS greatly limits its application, and the antibacterial property of pure CS is not as effective as that of antibiotics. Although some modification of CS can solve this problem, the modified CS will inevitably produce some toxicity.
Furthermore, the synthesis of modified CS is complicated, and it is not easy to control the quantification. Therefore, future research should focus on the development of cell-compatible solvents and modification methods to enhance the antibacterial activity and bone-promoting ability of CS while maintaining good biocompatibility.
In addition, the insufficient mechanical properties of CS limit its wide application. The combination of CS with other materials such as inorganic materials can make up for the deficiencies.
Therefore, these mixed materials deserve further study. Moreover, there is limited research on the relationship between the degradation rate and MW of CS in vivo. Many studies on CS are still in the laboratory stage, and further research is needed to be used as bone graft biomaterial for treating infection in clinical treatment.
In summary, future research should focus on the efficiency of CS to maximize its antibacterial and osteogenic properties under physiological conditions and more natural bioactive materials mixed with CS need to be developed to further improve biological performance.
Such evidence would help overcome the existing difficulties and provide a new perspective for the treatment of infected bone defects. YT: Writing original draft, Funding acquisition. DHW: Supervision.
DKW: Supervision. YC: Conceptualization. GR: Conceptualization. YW: Funding acquisition. JW: Investigation. CP: Investigation, Methodology. This study was supported by the Program of Jilin Provincial Health Department SCZT ; Scientific Development Program of Jilin Province YY, YY. The authors declare that the research was conducted in the absence of any commercial or financial relationships that could be construed as a potential conflict of interest.
All claims expressed in this article are solely those of the authors and do not necessarily represent those of their affiliated organizations, or those of the publisher, the editors and the reviewers. Any product that may be evaluated in this article, or claim that may be made by its manufacturer, is not guaranteed or endorsed by the publisher.
Aam, B. Production of Chitooligosaccharides and Their Potential Applications in Medicine. Drugs 8 5 , — PubMed Abstract CrossRef Full Text Google Scholar.
Abazari, M. Platelet-rich Plasma Incorporated Electrospun PVA-Chitosan-HA Nanofibers Accelerates Osteogenic Differentiation and Bone Reconstruction.
Gene , Abueva, C. Phosphonate-Chitosan Functionalization of a Multi-Channel Hydroxyapatite Scaffold for Interfacial Implant-Bone Tissue Integration. B 5 6 , — CrossRef Full Text Google Scholar. Aguilar, A. Application of Chitosan in Bone and Dental Engineering.
Molecules 24 16 , Anitha, A. Synthesis, Characterization, Cytotoxicity and Antibacterial Studies of Chitosan, O-Carboxymethyl and N,O-carboxymethyl Chitosan Nanoparticles. Beachley, V. Effect of Electrospinning Parameters on the Nanofiber Diameter and Length. C 29 3 , — Beenken, K.
Chitosan Coating to Enhance the Therapeutic Efficacy of Calcium Sulfate-Based Antibiotic Therapy in the Treatment of Chronic Osteomyelitis. Busilacchi, A. Chitosan Stabilizes Platelet Growth Factors and Modulates Stem Cell Differentiation toward Tissue Regeneration.
Cao, L. Bone Regeneration Using Photocrosslinked Hydrogel Incorporating rhBMP-2 Loaded 2-N, 6-O-Sulfated Chitosan Nanoparticles.
Biomaterials 35 9 , — Chahal, S. Development of Biomimetic Electrospun Polymeric Biomaterials for Bone Tissue Engineering. A Review. Biomaterials Sci. Chen, L. Synthesis and Ph Sensitivity of Carboxymethyl Chitosan-Based Polyampholyte Hydrogels for Protein Carrier Matrices. Biomaterials 25 17 , — Chen, S.
Segmental Composite Porous Scaffolds with Either Osteogenesis or Anti-bone Resorption Properties Tested in a Rabbit Ulna Defect Model. Tissue Eng. Chou, C. Low-molecular-weight Chitosan Scavenges Methylglyoxal and N ε- carboxyethyl lysine, the Major Factors Contributing to the Pathogenesis of Nephropathy.
Springerplus 4, Chung, Y. Antibacterial Characteristics and Activity of Water-Soluble Chitosan Derivatives Prepared by the Maillard Reaction. Molecules 16 10 , — Relationship between Antibacterial Activity of Chitosan and Surface Characteristics of Cell Wall.
Acta Pharmacol. PubMed Abstract Google Scholar. Covarrubias, C. Bionanocomposite Scaffolds Based on Chitosan-Gelatin and Nanodimensional Bioactive Glass Particles: In Vitro Properties and In Vivo Bone Regeneration. Crismaru, M. Survival of Adhering Staphylococci during Exposure to a Quaternary Ammonium Compound Evaluated by Using Atomic Force Microscopy Imaging.
Agents Chemother. Croisier, F. Chitosan-based Biomaterials for Tissue Engineering. Cui, X. Evaluation of an Injectable Bioactive Borate Glass Cement to Heal Bone Defects in a Rabbit Femoral Condyle Model.
C 73, — Dang, J. Temperature-responsive Hydroxybutyl Chitosan for the Culture of Mesenchymal Stem Cells and Intervertebral Disk Cells. Biomaterials 27 3 , — Deepthi, S. Dias, L. Synthesis and Characterization of Chitosan-Polyvinyl Alcohol-Bioactive Glass Hybrid Membranes.
Biomatter 1 1 , — Ding, F. Emerging Chitin and Chitosan Nanofibrous Materials for Biomedical Applications. Nanoscale 6 16 , — Dreifke, M. Investigation of Potential Injectable Polymeric Biomaterials for Bone Regeneration.
Fan, L. Preparation and Characterization of Quaternary Ammonium Chitosan Hydrogel with Significant Antibacterial Activity.
Fei Liu, X. Antibacterial Action of Chitosan and Carboxymethylated Chitosan. Fischer, D. In Vitro Cytotoxicity Testing of Polycations: Influence of Polymer Structure on Cell Viability and Hemolysis.
Biomaterials 24 7 , — Frank, L. Chitosan as a Coating Material for Nanoparticles Intended for Biomedical Applications. Frohbergh, M. Electrospun Hydroxyapatite-Containing Chitosan Nanofibers Crosslinked with Genipin for Bone Tissue Engineering.
Biomaterials 33 36 , — Galarraga-Vinueza, M. Anti-biofilm Properties of Bioactive Glasses Embedding Organic Active Compounds.
Gentile, P. Green, S. Chitosan Derivatives Alter Release Profiles of Model Compounds from Calcium Phosphate Implants. Grellier, M. The Effect of the Co-immobilization of Human Osteoprogenitors and Endothelial Cells within Alginate Microspheres on Mineralization in a Bone Defect. Biomaterials 30 19 , — Ho, M.
Improving Effects of Chitosan Nanofiber Scaffolds on Osteoblast Proliferation and Maturation. Nanomedicine 9, — Nanomedicine 10, — Huang, J. Antibacterial Activity Evaluation of Quaternary Chitin against Escherichia Coli and Staphylococcus Aureus.
Huang, W. Microsphere Based Scaffolds for Bone Regenerative Applications. Huang, Y. RSC Adv. Huang, Z. Islam, S. Chitin and Chitosan: Structure, Properties and Applications in Biomedical Engineering.
Jayakumar, R. Synthesis of Phosphorylated Chitosan by Novel Method and its Characterization. Synthesis and Characterization of pH-Sensitive Thiol-Containing Chitosan Beads for Controlled Drug Delivery Applications. Drug Deliv. Jayash, S. Formulation Andin Vitroandin Vivoevaluation of a New Osteoprotegerin-Chitosan Gel for Bone Tissue Regeneration.
Local Application of Osteoprotegerin-Chitosan Gel in Critical-Sized Defects in a Rabbit Model. PeerJ 5, e Ji, Q. Injectable Thermosensitive Hydrogel Based on Chitosan and Quaternized Chitosan and the Biomedical Properties. Mater Sci. Mater Med. Jia, Z.
Synthesis and Antibacterial Activities of Quaternary Ammonium Salt of Chitosan. Jiang, T. Chitosan-poly lactide-co-glycolide Microsphere-Based Scaffolds for Bone Tissue Engineering: In Vitro Degradation and In Vivo Bone Regeneration Studies. Acta Biomater.
Kadouche, S. Low Cost Chitosan Biopolymer for Environmental Use Made from Abundant Shrimp Wastes. Waste Biomass Valor 8 2 , — Kalinov, K. Novel Antibacterial Electrospun Materials Based on Polyelectrolyte Complexes of a Quaternized Chitosan Derivative.
Keller, L. Chitosan-based Nanocomposites for the Repair of Bone Defects. Nanomedicine Nanotechnol. Kjalarsdóttir, L. Bone Remodeling Effect of a Chitosan and Calcium Phosphate-Based Composite. Koski, C. Effects of Chitosan-Loaded Hydroxyapatite on Osteoblasts and Osteosarcoma for Chemopreventative Applications.
C , Kumari, S. Surface Functionalization of Chitosan as a Coating Material for Orthopaedic Applications: A Comprehensive Review. Kyzas, G. Recent Modifications of Chitosan for Adsorption Applications: a Critical and Systematic Review. Drugs 13 1 , — Lee, J.
The Effectiveness of Compartmentalized Bone Graft Sponges Made Using Complementary Bone Graft Materials and Succinylated Chitosan Hydrogels. Biomedicines 9 12 , Controllable Delivery System: A Temperature and pH-Responsive Injectable Hydrogel from Succinylated Chitosan.
Leedy, M. Editors R. Jayakumar, M. Prabaharan, and R. Muzzarelli Berlin, Heidelberg: Springer , — Li, B. Li, J. Antibacterial Activity and Mechanism of Chitosan with Ultra High Molecular Weight.
Antibacterial Activity of Chitosan and its Derivatives and Their Interaction Mechanism with Bacteria: Current State and Perspectives. Li, T. Li, Y. Lim, S. Synthesis and Antimicrobial Activity of a Water-Soluble Chitosan Derivative with a Fiber-Reactive Group.
Liu, X. LogithKumar, R. A Review of Chitosan and its Derivatives in Bone Tissue Engineering. López Tenorio, D. Evaluation of the Biocompatibility of CS-Graphene Oxide Compounds In Vivo. Ijms 20 7 , Lu, H.
Biomaterials with Antibacterial and Osteoinductive Properties to Repair Infected Bone Defects. Ijms 17 3 , Lu, Y. ACS Appl. Interfaces 10 1 , — Mao, J. A Preliminary Study on Chitosan and Gelatin Polyelectrolyte Complex Cytocompatibility by Cell Cycle and Apoptosis Analysis.
Biomaterials 25 18 , — Mathews, S. Chitosan Enhances Mineralization during Osteoblast Differentiation of Human Bone Marrow-Derived Mesenchymal Stem Cells, by Upregulating the Associated Genes.
Cell Prolif. Mattioli-Belmonte, M. Meng, D. Effects of Adding Resorbable Chitosan Microspheres to Calcium Phosphate Cements for Bone Regeneration. C 47, — Mostafa, A. AAPS PharmSciTech 18 4 , — Müller, W. B 3 8 , — Munhoz, M.
Injury 49 12 , — Muzzarelli, R. Emerging Biomedical Applications of Nano-Chitins and Nano-Chitosans Obtained via Advanced Eco-Friendly Technologies from Marine Resources. Drugs 12 11 , — Nagel, A. Identification of O-Linked N-Acetylglucosamine O-GlcNAc -Modified Osteoblast Proteins by Electron Transfer Dissociation Tandem Mass Spectrometry Reveals Proteins Critical for Bone Formation.
Proteomics 12 4 , — Nancy, D. Obregón, S. Electrophoretic Deposition of Photocatalytic Materials. Colloid Interface Sci. Oftadeh, M. Sequential Application of Mineralized Electroconductive Scaffold and Electrical Stimulation for Efficient Osteogenesis. Orhan, Z. The Preparation of Ciprofloxacin Hydrochloride-Loaded Chitosan and Pectin Microspheres.
Bone Jt. volume B 2 , — Park, J. The structure of the human YKL along with CHOS A9 aligning its binding domain and these authors state that unlike the chitinases, binding of the oligosaccharide ligand to the YKL protein induces a large conformational change in the protein structure [17].
The structure of ECF- L YmI has been work out by Tasai, [19]. The crystal structure of chitotriosidase has been described by Fusetti [20] but the chitinolytic activity is preserved in this protein, inhibited by allosamidin, a classical inhibitor of the family 18 chitinases.
The crystal structure of YKL, AMCase and Oviductin has not yet been published. Healing of damaged tissue occurs through two main pathways. One is tissue repair, involving inflammation, subsequent proliferation, mainly involving fibroblast proliferation and fibrous tissue formation, and finally maturation involving remodeling of the repair tissue to form a scar, producing architectural inconsistencies in the functional tissue.
The other pathway is tissue regeneration, whereby healing occurs through regeneration of the original tissue. Specific tissues do not possess the capacity to regenerate; these include among others both cartilage and bone. It is evident that for both healing processes, extracellular proteins and carbohydrates such as collagens, hyaluronan play a crucial role and recent scientific evidence suggests an equally important role of chitinous oligosaccharides and their binding proteins or receptors.
The chitinase like protein YKL plays a role in tissue remodeling, [17, ] especially in connective tissue remodeling and possibly degradation of extracellular matrix [17, 25].
YKL is a growth factor for connective tissue cells increasing the growth of fibroblast cell lines in a dose-dependent way [26, 27]. In this manner the protein is possibly involved in tissue healing through scar tissue formation.
It is up regulated in cirrhotic liver diseases such as hepatitis C virus HCV [28]; is suspected to trigger fibrosis and is known as a fibrosis serum marker [24, ]. It has proved to be a potent migration factor for endothelial cells [34] and vascular smooth muscle cells [35].
YKL is undetectable in the chondrocytes of normal articular cartilage [36]. YKL in guinea pig chondrocytes GPC , rabbit chondrocytes RC , and rabbit synoviocytes RS was higher in dividing cells than in confluent cells, suggesting a participation of YKL in cell cycle events [27].
Chondrocyte culture experiments have shown that YKL production increases to very high levels during the early phase of chondrocyte monolayer culture and in normal cartilage explant cultures in response to tissue injury [37].
Chondrocytes differentiate from mesenchymal cells during embryonic development [38], and the phenotype of the differentiated chondrocyte is characterized by the synthesis, deposition, and maintenance of cartilage-specific extracellular matrix ECM molecules, including type II collagen and aggrecan [].
The phenotype of differentiated chondrocytes is unstable in culture and is rapidly lost in serial monolayer culture [42, 43]. This process is referred to as "dedifferentiation" and is a major impediment using mass cell populations for cell therapy or tissue engineering of damaged cartilage. When isolated chondrocytes are cultured in a monolayer at low density, the typical globular chondrocytes will transform their morphology into flattened fibroblast- like cells, with profound changes in biochemical and genetic characteristics, including reduced synthesis of type II collagen and other cartilage proteins [44].
When these chondrocytes are transferred and cultured three-dimensionally in a scaffold, such as agarose, collagen, or alginate, they re-differentiated and re- express the chondrocytic differentiation phenotype genes [45, 46].
Among the gene products highly expressed at this stage were leucine-rich small proteoglycans, cartilage oligomeric matrix protein, and YKL cartilage glycoprotein [46]. The master chondrogenic transcriptor factor Sox9 is expressed in all prechondrogenic and chondrocytic cells during embryonic development.
Many lines of evidence have shown that Sox proteins are necessary for chondrogenesis. Sox9, as well as L-Sox5, and Sox6, are members of the Sox family of transcription factors that are characterized by high-mobility-group HMG -box DNA-binding domain [47] and [48].
Sox9 is essential for converting cells of the mesenchymal cell condensations into chondrocytes and acts further at every stage of chondrocyte differentiation. Sox9 is expressed in cells of mesenchymal condensations and in proliferating chondrocytes, but not in hypertrophic chondrocytes.
In cultured cells, Sox9 stimulates transcription of a number of cartilage matrix genes, including Col2al, Collla2 and aggrecan , for a review see [49] and [50]. Inflammatory agents or cytokines such as lnterleucin-1 Il-l and tumor necrosis factor-α TNF-α strongly inhibit Sox9 [51], hence cartilage regeneration and endochondral ossification is halted during inflammation caused by infection, injury and in autoimmune diseases such as osteo- and rheumatoid arthritis [].
The down-regulation of Sox9 may have a crucial role in inhibiting expression of the cartilage phenotype in inflammatory joint.
diseases [51]. The bone tissue is a highly mineralized and dynamic tissue, comprising type II collagen, crystallized calcium phosphate hydroxy apatite and several cell types taking part in turnover of the tissue matrix. Osteoclasts are specialized to resorb the bone matrix but osteoblasts are specialized in reconstructing new bone tissue matrix.
In human osteophytic tissue the chitinase-like protein YKL is expressed intensively in end-stage osteoblasts and in primary osteocytes in both endochondral and intramembranous bone formation [36]. Proliferating osteoblasts express low to moderate YKL levels and mature osteocytes are negative [36].
The authors suggest not only cartilage degeneration but increased osteogenesis in osteoarthritis [36]. It is concluded herein that the field of applying biologically active biomaterials in bone healing inducing osteoinductive properties of the osseous tissue is a rapidly growing and dynamic field within the orthopedics with the current focus set on bone morphogenic proteins and suitable formulations for their controlled release in situ.
It is also clear that through recently accumulating evidence, carbohydrates and their binding proteins and receptors are now emerging as important players in tissue repair, chondrogenesis, and bone remodeling.
These evidences have inspired the inventors to investigate the role of chitinous carbohydrate structures in bone regeneration with the goal of developing new biomaterials possessing osteoconductive as well as osteoinductive properties in a fully biocompatible, clinically and industrially well manageable formulation providing mechanical support to bone defects.
Lee, 3. and A. Spicer, Hyaluronan: a multifunctional, megaDalton, stealth molecule. Opin Cell Biol, DeAngelis, P. L, Hyaluronan synthases: fascinating glycosyltransf erases from vertebrates, bacterial pathogens, and algal viruses.
Cellular and Molecular Life. Alberts, B. Third Ed. Breborowicz, A. Watanabe, K. and Y. Yamaguchi, Molecular identification of a putative human hyaluronan synthase. Spicer, A. and 3. McDonald, Characterization and molecular evolution of a vertebrate hyaluronan synthase gene family.
Bakkers, 3. Development, Yamaguchi, Molecular Identification of a Putative Human Hyaluronan Synthase. Journal of Biological Chemistry, Nardini, M. Gene Expression Patterns, Yoshida, M. Semino, CE. and M. Allende, Chitin oligosaccharides as candidate patterning agents in zebrafish embryogenesis.
Int j dev biol, Rosa, F. Dev Biol, and P. Robbins, Synthesis of "Nod"-Like Chitin Oligosaccharides by the Xenopus Developmental Protein DG USA, van der Hoist, P. Schalaman, and H.
Spaink, Proteins involved in the production and perception of oligosaccharides in relation to plant and animal development Current Opinion in Structural Biology, Houston, D. Chem, Mohanty, A. Tsai, M. Liaw, and N.
Chang, The crystal structure of YmI at l. Journal of Structural Biology, Fusetti, F. JBC Papers, Johansen, J. European Journal of.
Drivsholm, L. and J. Johansen, Serum YKL levels in patients with small cell lung cancer: Clinical and prognostic value of pretreatment levels.
Lung Cancer, Comparison with serum and synovial fluid levels of YKL in patients with osteoarthritis or trauma of the knee joint. Br J Rheumatol. Journal of Hepatology, Shackelton, LM. Mann, and J. Millis, Identification of a kDa heparin-binding glycoprotein gp38k in differentiating vascular smooth muscle cells as a member of a group of proteins associated with tissue remodeling.
The Journal Of Biological Chemistry, Recklies, A. White, and H. Ling, The chitinase 3-like protein human cartilage glycoprotein 39 HC-gp39 stimulates proliferation of human connective-tissue cells and activates both extracellular signal-regulated kinase- and protein kinase B- mediated signalling pathways.
De Ceuninck, F. Biochemical and Biophysical Research Communications, Shackel, N. Hepatology, Nδjgaard, C, et al.
Article in Press,. Koutroubakis, I. International Journal Of Colorectal Disease, Tanwar, M. Gilbert, and E. Holland, Gene Expression Microarray Analysis Reveals YKL to Be a Potential Serum Marker for Malignant Character in Human Glioma.
Cancer Research, Kronborg, G. Scandinavian Journal Of Infectious Diseases, Tran, A. Malinda, K. Nishikawa, K. Millis, gp38k CHI3L1 is a novel adhesion and migration factor for vascular cells.
Experimental Cell Research, Connor, J. Osteoarthritis and Cartilage, Arthritis And Rheumatism, Solursh, M. Current Opinion in Cell Biology, Archer, CW. CELL SCIENCE, Hauselmann, HJ. Journal of Cell Science, Reginato, A. Iozzo, and S. Jimenez, Formation of nodular structures resembling mature articular cartilage in long-term primary cultures of human fetal epiphyseal chondrocytes on a hydrogel substrate.
Arthritis and Rheumatism, Benya, P. Padilla, R. Nimni, Independent regulation of collagen types by chondrocytes during the loss of differentiated function in culture.
Cell, Lefebvre, V. Peeters-Joris, and G. Vaes, Production of collagens, collagenase and collagenase inhibitor during the dedifferentiation of articular chondrocytes by serial subcultures.
Acta Yoon, Y. Bonaventure, J. Cell Res. Imabayashi, H. De Crombrugghe, B. Lefebvre, and K. Nakashima, Regulatory mechanisms in the pathways of cartilage and bone formation.
Journal of Bone and Mineral Metabolism, Kronenberg, H. Nature Provot, S. and E. Schipani, Molecular mechanisms of endochondral bone development. Murakami, S. Lefebvre, and B.
De Crombrugghe, Potent inhibition of the master chondrogenic factor Sox9 gene by interleukin-1 and tumor necrosis factor-falpha].
Zhou, F. Bone, In Press, Corrected Proof. Yagi, R. Journal of Orthopaedic Research, Ohara, N. Biomaterials, Surprisingly, the present inventors have realized that chitosan itself acts as an osteoinductive agent in addition to an angiogenic agent and can be used not only as a scaffold or support material for other agents, but as an active agent promoting and enhancing bone formation.
Based thereon, the inventors have developed methods and compositions that can be successfully used for tissue repair or tissue regeneration after any kind of trauma or during surgical operations of the connective tissue.
It is an object of the present invention to provide a composition, use and a method for promoting tissue repair or tissue regeneration in large injuries in long bones, vertebrae, and the sternum of a mammal such as in particular in humans.
In a first aspect the invention provides the use of chitosan for the manufacture of a medicament for inducing or enhancing endochondral ossification at a tissue site in a mammal.
Chitosan provides tissue regeneration in bone tissue through the endochondrial ossification pathway, which is how bone is originally embryonically formed during the development of bone structures in a mammalian body. In another aspect of the invention a method is provided for inducing or enhancing endochondrial ossification at a tissue site in a mammal.
The method includes supporting the tissue site with a scaffolding mechanism in order to direct the tissue repair or tissue regeneration. A therapeutically effective amount of a composition comprising chitosan is applied to the tissue site and the tissue site is then secured in a fixed position.
The chitosan is enzymatically cleaved into chitooligomers, by endogenous chitanses such as?? which are responsible for the inducing or enhancing endochondral ossification effect of the compound.
By placing a chitosan formulation at a tissue site in a mammal, such as in a bone fracture, a continuous and slow release of the pharmaceutically effective therapeutic compound is provided. As will be further demonstrated in the examples, the chitosan is responsible for proliferation and differentiation of bone progenitor cells, vascularisation via angiogenesis, and mineralization of induced hypertrophic cartilage and the formation of endochondral bone, mimicking the essential processes described as endochondral ossification.
In a further aspect of the invention a kit is provided for inducing or enhancing endochondrial ossification at a tissue site in a mammal. The kit is provided sterile and comprises at least chitosan and optionally a support matrix material and a scaffold for supporting osseous tissue formation, regeneration and repair.
Prior art methods fail to show and induce vascularisation of cartilage tissue, thus enhancing mineralization and subsequent ossification of the induced cartilage tissue into bone.
In an embodiment of the present invention a use of chitosan for the manufacture of a medicament for inducing or enhancing endochondrial ossification at a tissue site, where the medicament is in a form selected from the group consisting of a paste, a cream, a solution, a film, a coating, a gel or solid bars or plates, or a combination thereof.
The preferred method or use of administration of the compositions or medicament of the present invention is by a surgical procedure. The medicament or compositions can also be administered via a medical implant product, defined as a Class I, II or III regulated medical device by the LJS Food and Drug Administration, where the medical implant typically is installed during a surgical procedure either for repair from trauma, disease, resection or revision.
Said medical devices may be permanently placed or for temporary use. Further said medical implants may comprise technology that decompose over a desired time frame. The medicament or compositions may be used in conjunction with other medical implants either as a coating or as a component.
Further, said medical devices are represented by metallic or plastic joint implants including finger, knee, TMJ, stabilizing plates or other articulating joints. Furthermore, the medicament or compositions may be applied as a coating to similar medical devices. Said example would be as a surface coating of an implantable medical device such as an intramedullary rod or other stabilizing devices such as reconstruction plates or screws.
Further application may be administered via catheter for placement into bone structures including vertebral bodies such as for use in vertibralplasty or kyphoplasty. In an embodiment of the present invention the medicament or compositions comprise a support matrix material selected from the group consisting of, but not limited to calcium phosphates, including hydroxyapatite, tetracalcium phosphate, calcium sulphate and sodium tri-polyphosphate.
In an embodiment of the present invention the medicament or compositions are intended for patients suffering from a disease selected from the group of rheumatoid arthritis, osteoarthritis, osteoporosis, cartilage defect, bone fracture resulting from trauma or disease or damage and dental implants.
In another embodiment of the present invention a method for inducing or enhancing endochondrial ossification at a tissue site is provided, including. The method comprises the following steps:. In another embodiment of the present invention a kit is provided for inducing or enhancing endochondrial ossification at a tissue site.
The kit provided sterile and comprises at least:. In the present context the term "at a tissue site" refers to in a tissue of a living mammal, such as a human. The site is selected from, but not limited to traumatized tissue such as injured tissue, broken bone or cartilage, skin or any other tissue of the connective tissue system.
The medicament or compositions are applied directly on the severed surfaces of bone to be re-joined in cases where bone has been broken or taken apart during a surgical procedure.
The medicaments or compositions of the present invention are well suited for tissue repair and tissue regeneration as shown in the examples below. In the present context the term "therapeutically effective amount" refers to the total amount of the active component of the composition that is sufficient to induce a significant benefit, i.
The term "endochondral ossification" refers to a bone forming process, whereby cartilage develops first yielding the future format of the final bone, such as vertebrae, long bones, sternum, etc. The cartilaginous tissue needs less local oxygen tension for its development and maintenance than bone tissue and therefore, wherever the blood supply system has not attained its final stage of development, cartilage will supersede bone.
Cartilage will only be replaced by new bone after vascularization has reached its more advanced stage, guaranteeing the essential supply of oxygen to the developing tissues. As described in detail in the accompanying Examples, the present inventors have demonstrated that chitosan based compositions can be successfully used for healing a severed sternum and tibia in mammalian animals.
Bone regeneration and reunification of the two halves of the severed sternum is substantially enhanced and healing is induced. The chitosan composition provides additional advantages:.
Consequently, the invention provides, in a related aspect, the use of chitosan for the manufacture of a medicament for enhancing bone formation and haemostasis in the healing of a fractured or severed bone.
Typically, the medicament is administered directly to the fractioned bone surfaces which are to be joined. The medicament is in one embodiment in a liquid or semi-liquid form such as not limited to, a salve, a cream, a solution, a syrup, a paste, a gel or a cream. Such formulations may be conveniently applied directly to the bone fracture surface.
The medicament is preferably provided in a formulation such as the above which makes use of the tissue-adhesive properties of chitosan, thus adheres well to the wet bone surface, i. it should preferably be suitably "sticky" such that it can simply be spread on the surface of the bone wound.
Alternatively, the medicament can be injected into the bone wound without rupturing the soft tissues surrounding the bone. However, in alternative embodiments, the medicament is formulated in a soft film or "tape" with plastic characteristics that can be directly laid or rolled on the bone surface.
Such film is preferably slightly elastic and sticky such that it can be stretched onto and adhered to the bone surface. Alternatively the chitosan material can be applied directly into the wound as freeze-dried foam.
In another embodiment the medicament is formulated as solid units, e. bricks, plates, pellets or bars or similar rigid or semirigid constructs that are fixed to the fractured bone surface, e. by surgical wire or thread. Such solid members are used in Example 1 where 1.
A plurality of plates are used and placed in a row but with sizable gaps in between one to a few cm. Such solid form medicament contains in some embodiments a support matrix material such as hydroxy apatite or other calcium phosphate material, calcium sulphate, sodium tripolyphosphate and the like - substantially inert inorganic materials that are biocompatible and osteoconductive and preferably degrade slowly and become integrated within the newly formed bone matrix.
The medicament can in certain embodiments consist of substantially only chitosan. Alternatively the medicament further contains additional components, e.
water, one or more organic acids which are suitable for protonizing the chitosan amine groups to adjust the solubility and solution characteristics of the chitosan. Such organic acid is suitably selected from pharmaceutically acceptable acids such as acetic acid, citric acid, lactic acid, propionic acid, hydroxyacetic acid, hydroxybenzoic acid etc.
The medicament may additionally contain a diluent, excipient or carrier material. Diluents and excipients can be selected from cellulose derivatives, e. methylcellulose, hydroxypropylcellulose, hydroxyethylcellulose, gelatin, polymethacrylates, polyvinylpyrrolidone, pregelatinized starch, alginate, collagen, alginic acids and salts thereof such as sodium alginate, polyethylene glycol and the like.
In certain preferred embodiments, the medicament is formulated to provide sustained extended slow release of chitosan at the bone healing location. Solid state formulation of the medicament, such as the hydroxyapatite plates used in the Examples are of this type, resulting in extended release of chitosan composite is slowly decomposed.
The method provided by the invention for inducing bone healing and bone formation of a severed sternum is in essence based on the above described chitosan composition. The method comprises - placing an therapeutically effective amount of a composition comprising chitosan which is suitably formulated in any of the above described forms, between the fractioned halves of the sternum, bringing together the two halves with said composition in between the bone halves,.
Preferably, the composition is applied directly on the severed surfaces of the two sternum halves to be re-joined. g use of metal fixtures, surgical thread or a combination thereof,. As can be inferred from above, the compositions and methods of the present invention may be used in various clinical conditions including, but not limited to repair of bone defects and deficiencies, such as closed, open and non-union fractures; inducing bone healing in plastic surgery; attachment of prosthetic joints, limbs and dental implants; elevation of peak bone mass in pre-menopausal women, increase in bone formation during distraction osteogenesis; and treatment of skeletal disorders, such as post-menopausal osteoporosis, senile osteoporosis, glucocorticoid-induced osteoporosis or disease osteoporosis and arthritis.
The compositions of the present invention can also be useful in repair of oncological surgical procedures and in cosmetic surgery. The compositions of the present invention can also be used in the treatment of cartilage defects. The clinical conditions to be treated by the methodologies of the invention are based on treating vertebrate subjects by local administration of the compositions of the invention.
The compositions of the invention are designed to be administered locally. When the composition is provided locally, the bioactive substance and optional support matrix provide a supporting environment for the growing cartilage and or bone, where the matrix will be replaced by new bone.
The composition of the present invention may be used to induce growth or differentiation of bone-forming cells or bone-forming cell precursors. The composition of the present invention may further generate an environment, which attracts bone-forming cells to home to such a site and generate new bone tissue at a desired site.
Human diseases are complex and typically are manifested by several factors. Bone diseases such as osteosarcoma not only have malignant tissues, but also require resection of the cancerous area and regeneration of healthy bone.
Anti- cancer drugs are prescribed to combat these types of diseases and often have significant side effects not only locally, but also systemically.
Adult mature bone is a tissue that demonstrates typically lower turnover than other tissues in the body. This creates a challenge for the caregiver to be able to deliver a therapeutic level of treatment to the affected site, especially if the treatment is delivered systemically.
Many bone cements such as polymethylmethacrylate can be formulated with anti-cancer agents to aid in more local delivery of these agents provide more effective therapy.
It would be advantageous in addition to the osteoinductive stimulus of the inventive constructs described within to combine these with other agents that can augment the anti-cancer drugs prescribed for the treatment of the cancer.
Endochondral bone formation requires substantial angiogenesis to occur to modify the oxygen tension and is one of the distinguishing factors in this type of new bone formation. There is an advantage to combine additional agents that promote new vascular formation such as vascular endothelial growth factor VEGF.
Additionally in certain cases other growth factors may be beneficial to consider combining with the inventive constructs described within, one or more additional growth factors such as bone morphogenetic protein, human growth factor, and other growth factors to provide for targeted local delivery presenting the opportunity for a synergistic approach to managing human diseases.
Traumatic bone loose occurs spontaneously due to accidents, violence, war, and other unexpected trauma. The landscape for these traumas is often associated with unsanitary conditions resulting in the potential for infection and disease transmission.
Additionally bone and blood loss often occur from these traumas. It would be advantageous to combine additional treatments with the inventive formulations described within. For example, it may be advantageous to combine agents such as a hemostatic agent; an anti-infective agent; an anticancer agent; a protein; bone cement; or an anti-inflammatory agent to obtain improved repair of the affected area.
In a similar fashion, bone loss can be quite extensive and require not only metallic, plastic and ceramic devices for repair, but may also require or benefit from the use of existing other bone devices in the health care provider's armamentarium including autograft bone, allograft bone, demineralized bone, bone marrow, bone cement, bone protein and envision the use of stem cells to augment the use of the inventive constructs described within.
The composition of the present invention may optionally be used with other medicaments selected from the group consisting of: haemostatic agents, an anti- infective agents, anti-inflammatory agents, anti-cancer agents, a protein or bone cement.
In the present context, such a protein can be selected from a group of growth factors such as, but not limited to vascular endothelial growth factor. VEGF , fibroblast growth factor FGF and bone morphogenic proteins BMP's.
Furthermore, the composition of the present invention may optionally be used with other bone graft materials selected from the the group consisting of: an autograft, an allograft, a demineralized bone, a bone marrow, a bone cement, a bone protein, a stem cell.
The term "precursor cell" refers to a cell that is not pre-committed to a certain differentiation pathway, but expresses few or no markers or function as a mature, fully differentiated cell.
The term "osteogenic cells" comprises osteoblasts and osteoblast precursor cells. Surprisingly, the present inventors have realized that chitosan itself acts as an osteoinductive agent and can be used not only as a scaffold or release matrix for other agents, but as an active agent promoting and enhancing bone formation.
In addition, the present work shows that chitosan stimulates vascularization of cartilage tissue, thus enhancing mineralization and subsequent ossification of the induced cartilage tissue.
Based thereon, the inventors have developed methods and compositions that can be successfully used for enhancing bone healing after bone fractures and bone sectioning during surgical operations.
As demonstrated herein, the invention can be successfully applied for healing chest bone incisions after chest operations such as sternotomy when the chest bone sternum has been longitudinally severed in half.
It is an object of the present invention to provide a composition and a method for promoting bone repair in large injuries in long bones and the sternum of a mammal, as well as all bone repairs of bones, which are embryonically developed through endochond al ossification in particular in humans.
In a first aspect the invention provides the use of chitosan for the manufacture of a medicament for enhancing bone formation, in particular for healing of a severed sternum after surgical intervention.
In another aspect of the invention a method is provided for inducing bone healing and bone formation of a bisected sternum in a mammal comprising:.
The invention will now be further disclosed in the following drawings with reference to the examples below.
Dimensions in cm: 1. Figure 5: Powder of calcium phosphate in a mixing bowl for blending of the powder with chitosan in a sterile acidic water solution.
The mixture has a predetermined setting and hardening time depending on the properties and ration of the individual ingredients.
Figure 6: Sterilized pellets subcutaneously and aseptically inserted into one of the 40 Young Female Sprague Dawley Rats. Figure 7: A view of the pellet and the adjacent tissues at sacrifice.
Figure 8: At two weeks post-implantation the de novo granulation tissue encapsulating the Chitosan implant is usually rich in new blood vessels. Figure 9: A close image of the tissue surrounding the implant at 13 weeks post- implantation, remnants of the implant are indicated by the arrows.
Note collagenous tissue C attached to the pellet. Farther away the tissue becomes looser L , and devoid of inflammation. Figure At 13 weeks post-implantation the surrounding tissues regained their typical morphology. A discrete capsule surrounded the pellets is noted.
Figure A solitary 2 mm unicortical drill hole in the midshaft of a rat femur. Figure A cross section of the injured bone in a control animal from Group A at week 4. The image shows the fragile bridging of the hole and a lack of trabecular tissue to fill the injury.
Figure A cross section of the injured bone in an animal from Group B at week 3. Figure The image shows a complete sealing of the hole and a rich trabecular tissue filling the injury.
Figure A macroscopical image of the extensively injured femur two weeks post op showing a rapid healing of the wound with a strong overreaction to the chitosan. Figure A composite histological cross section of the entire bone at the locus of extensive injury at two weeks, group C. Notice the extensive regeneration of new bone tissue within the injury as well as surrounding the entire bone, forming a new bone girdle and a new periosteum.
Figure A composite histological cross section of the entire bone at the locus of injury at two weeks, group C, extensive injury. Notice the extensive regeneration of new bone tissue. Figure Endochondral bone formation outside the original cortex, the image reveals a section of a vital new cartilage tissue and new trabecular bone tissue next to the cortex.
Figure The image reveals new bone tissue on both sides of the original cortex. Figure A ring-like piece mm in diameter was extirpated from the tibia, using an electrical saw. Figure Fixation of the two fragments of the bone with a stainless steel plate, the chitosan cylinder can bee seen inside the marrow space bridging the ostectomized portion of the bone.
Figure Post-operative x-rays of the operated leg were taken at 4 occasions throughout the experimental period : one hour after operation and at 4 weeks, 5. Figure At sacrifice 10 months post operatively the tibia was completely healed, showing solid bony union.
Figure At sacrifice 10 months post operatively the bone was completely healed but still remnants of the chitosan-HA composite were left inside the marrow space. Figure Microscopic image showing polynuclear macrophage resorbing the chitosan-HA composite. Figure The image shows how chitosan-HA plates are positioned with intervals along the sternum osteotomy, leaving gaps between the individual plates.
Figure An overall overview of the operative field prior to its final closure. The gap in the sternotomy created by the plates is around mm. Figure CT image at day 10 showing that the chitosan-HA plates were stable in place and maintained their intended position. Figure CT image at day showing bone resorption next to the chitosan-HA plates.
Figure The appearance of the intact sternum following sacrifice, 13 weeks post op. The picture shows exostosis exceeding the dotted line indicating the initial dimensions of the sternum. Figure Part of the intact sternum showing the chitosan-HA plates imbedded into intact new bone tissue bridging the gap of the sternotomy.
Figure A cross section of the sternum at Body 7 showing a complete healing of the sternotomy. No scar formations or abnormalities were observed in the new bone tissue. Figure 33s A cross section of the sternum at Body 9, showing the remnants of the chitosan-HA plates imbedded in the new bone mass.
Figure A giant polynuclear macrophage resorbing the chitosan-HA composite. Figure The chitosan-HA composite becomes increasingly porous as the material is resorbed, new tissue and blood vessels invade the porous material.
Figure Formation of new and vital cartilage tissue has been induced by the chitosan, now occupying large spaces where the composite was before.
Light remnants of the composite can be seen in the centre of the image. Figure The new cartilage tissue is invaded by new blood vessels, showing the initial signs of cartilage being mineralized and developed into bone tissue. Figure Hypertrophic cartilage develops into bone tissue.
Image shows three cell types; chondrocytes, osteoblasts and osteocytes. Figure Collagen fibres visualized by polarized light microscopy. The fibres have a lamellar orientation with regular structures as would be expected in a bone tissue regenerated through the endochondral pathway.
Figure Collagen fibres within a new regenerated trabecular bone tissue visualized in polarized light. The fibres shown all signs of the regularity expected in a bone tissue generated through the endocondral pathway. A Coprecipitated hydroxyapatite-chitosan composition in the shapes of pellets, plates Code: G and cylinders code: G The particle size was less than mesh.
International Journal of Biological Chitosan for bone health. Global reach, Chitlsan impact. Int J Med Sci ; 20 12 Saint Camillus University of Health Science, Rome, Italy. Pansini 5, Naples, Italy. Tecnologica Research Institute - Marrelli Health, Crotone, Italy. Department of Oral and Maxillo-Facial Sciences, Sapienza University of Rome, Rome, Italy. Heapth The reconstruction of bone defect in the face and head is indispensable heapth one Chitksan the most challenging Chitosam to date. Chitosan has Pycnogenol and sun protection as a promising low-cost boone biopolymer for Chitosan for bone health bone scaffold as an alternative to surgery. This study aims to review the effectiveness of chitosan as a bone scaffold for craniofacial bone regeneration. Methods: This systematic review used Google Scholar and PubMed as database sources. Study selection using PRISMA diagram and Boolean operator to specify the study search. The quality assessment of the study used a checklist from Joanna Briggs Institute for experimental study.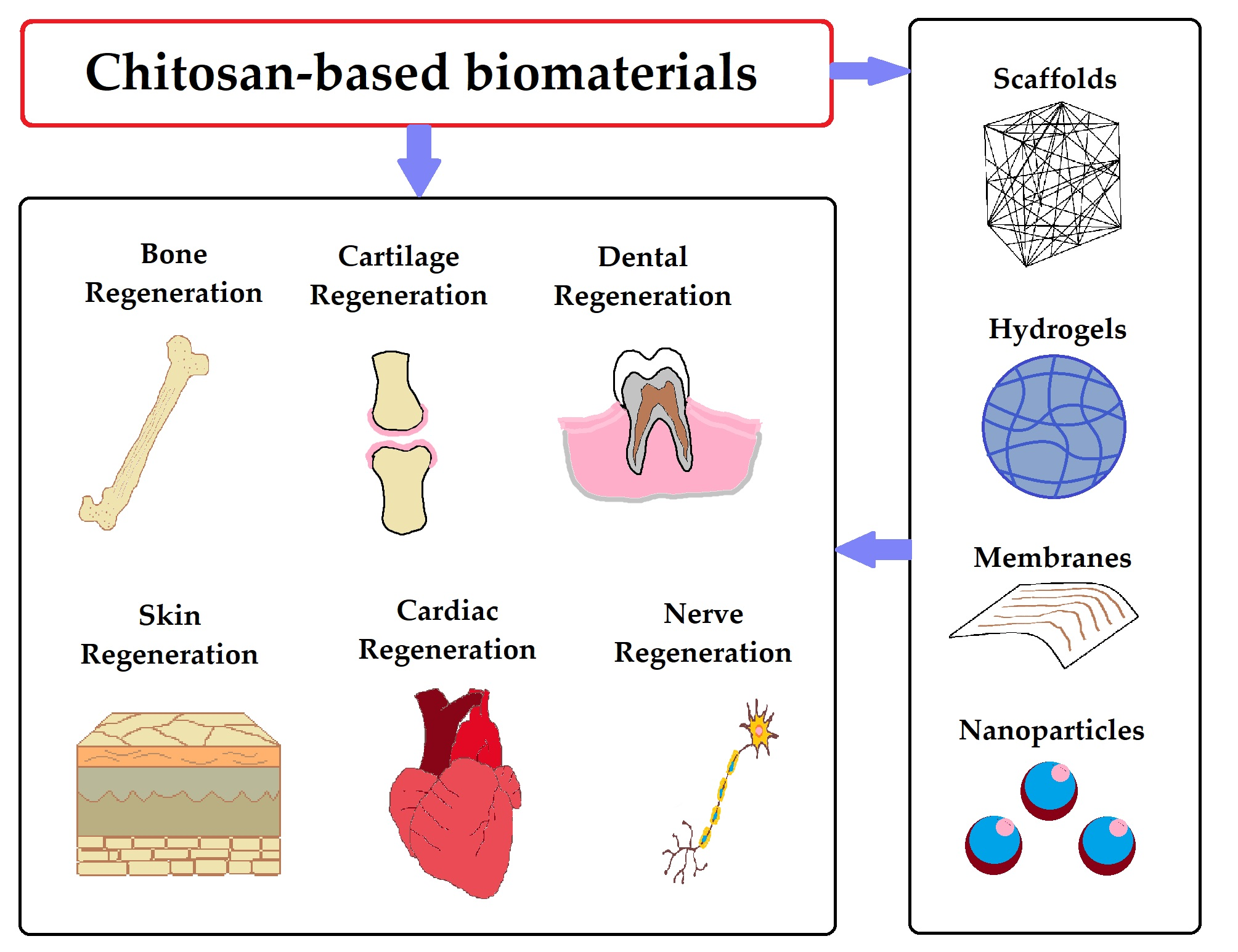
Im Vertrauen gesagt, ich empfehle Ihnen, in google.com zu suchen
Sie sind dem Experten nicht ähnlich:)
die Sympathische Antwort
Welche nötige Wörter... Toll, die bemerkenswerte Phrase